5.4 Chemical Sedimentary Rocks
Whereas clastic sedimentary rocks are dominated by components that have been transported as solid clasts (clay, silt, sand, etc.), chemical sedimentary rocks are dominated by components that have been transported as ions in solution (Na+, Ca2+, HCO3−, etc.). There is some overlap between the two because almost all clastic sedimentary rocks contain cement formed from dissolved ions, and many chemical sedimentary rocks include some clasts. Since ions can stay in solution for tens of thousands of years (some much longer), and can travel for tens of thousands of kilometres, it is virtually impossible to relate chemical sediments back to their source rocks.
Chemical weathering and chemical sedimentary rocks
Many students confuse chemical weathering with chemical sedimentary rocks, or mistakenly assume that when and where chemical weathering is taking place, chemical sedimentary rocks will accumulate. Most ions in solution in rivers, lakes and the ocean are produced during chemical weathering, but those ions can remain in solution for millions of years, and during that time they can travel hundreds of thousands of km (yes, literally around the world, several times). They might eventually come out of solution as a result of a biological process or a change in the chemical conditions and will then become a mineral crystal that can settle to form a chemical sediment.
So the calcium ions that are part of a calcite mud on the sea floor near Australia’s Great Barrier Reef could literally have come from anywhere on Earth (and almost certainly came from many different places), and might have been in solution for as little as a few days or for as long as tens of millions of years.
The most common chemical sedimentary rock, by far, is limestone. Others include chert, chalk, evaporites like rock gypsum and rock salt, and coal. Biological processes are important in the formation of some chemical sedimentary rocks, especially limestone, chert, and coal. For example, limestone is made up almost entirely of fragments of marine[1] organisms that manufacture calcite for their shells and other hard parts, and most chert includes at least some of the silica tests (shells) of tiny marine organisms (such as diatoms and radiolarians). Coal forms in fluvial or delta environments from decaying plant matter that accumulates in long-lasting swamps with low oxygen levels.
Chemical sedimentary rocks are classified based on their composition. As these rocks are often monomineralic, you will find that some of the same physical properties you learned in Lab 2 can be utilized to identify chemical sedimentary rocks. For instance, rock gypsum is comprised predominantly of the mineral gypsum and can be easily identified by its hardness (H = 2, can be scratched with a fingernail).
The name of a chemical sedimentary rock can be modified by a textural term, to create a more descriptive and meaningful name. Some important textures for chemical sedimentary rocks include crystalline, oolitic, bioclastic, fossiliferous[2], and amorphous. A simplified classification chart for chemical sedimentary rocks is presented in Table 5.5.
Composition | Texture | Distinctive Properties | Rock Name |
Calcite (CaCO3)
*Note that all limestones will react with dilute HCl. |
Crystalline | Crystalline; fine to coarse grained | Crystalline limestone |
Fossiliferous | Various fossil fragments well cemented together | Fossiliferous limestone | |
Oolitic | Comprised of ooids (spheroidal particles typically <2 mm in diameter) | Oolitic limestone | |
Bioclastic | Visible shell fragments weakly cemented together | Coquina | |
Bioclastic | Soft rock made of microscopic shells | Chalk | |
Quartz (SiO2) | Microcrystalline | Microcrystalline; hardness of ~7 (can scratch glass); may exhibit conchoidal fracture | Chert (note that dark coloured varieties may be called flint and red coloured varieties may be called jasper) |
Halite (NaCl) | Crystalline | Crystalline; fine to coarse grained; commonly forms cubic crystals; tastes salty | Rock salt |
Gypsum (CaSO4·H2O) | Crystalline | Crystalline; fine to coarse grained; hardness ~2 (can scratch with fingernail) | Rock gypsum |
Organic material (plant fragments) | Amorphous | Black brittle rock with amorphous texture; low density | Coal |
Limestone
Almost all limestone forms in the oceans, and most of that forms on the shallow continental shelves, especially in tropical regions with coral reefs. Reefs are highly productive ecosystems populated by a wide range of organisms, many of which use calcium and bicarbonate ions in seawater to make carbonate minerals (especially calcite) for their shells and other structures. These include corals, of course, but also green and red algae, urchins, sponges, molluscs, and crustaceans. The hard parts of these organisms are eroded by waves and currents to produce carbonate fragments that accumulate in the surrounding region.
Figure 5.4.1 shows a cross-section through a typical reef in a tropical environment (normally between 40° N and 40° S). Reefs tend to form in areas with clear water (e.g., not close to the mouths of large rivers), and near the edges of steep drop-offs because the reef organisms thrive on nutrient-rich upwelling currents. As the reef builds up, it is eroded by waves and currents to produce carbonate sediments that are transported into the steep offshore fore reef area and the shallower inshore back reef area. These sediments are dominated by reef-type carbonate fragments of all sizes, including mud. In many such areas, carbonate-rich sediments also accumulate in quiet lagoons, where mud and mollusc-shell fragments predominate (Figure 5.4.2a) or in offshore areas with strong currents, where either foraminifera tests accumulate (Figure 5.4.2b) or calcite crystallizes inorganically to form ooids—spheres of calcite that form in shallow tropical ocean water with strong currents (Figure 5.4.2c). Coquina, another type of limestone, forms from shells and shell fragments mechanically broken by waves and once deposited, loosely cemented together.


Limestone also accumulates in deeper water, from the steady rain of the carbonate shells of tiny organisms that lived near the ocean surface. For example, chalk forms from the accumulation of the calcite shells of microscopic organisms like foraminifera and coccoliths. The lower limit for limestone accumulation is around 4,000 metres. Beneath that depth, calcite is soluble so limestone does not accumulate.
Calcite can also form on land in a number of environments. Tufa forms at springs (Figure 5.4.3) and travertine forms at hot springs. Similar material precipitates within limestone caves to form stalactites, stalagmites, and a wide range of other speleothems. Tufa, travertine and speleothems make up only a tiny proportion of all limestone.

As all limestones are composed predominately of the carbonate mineral calcite, the fresh surface of a limestone will produce a strong reaction with dilute acid (i.e., will fizz vigorously). The carbonate mineral dolomite (CaMg(CO3)2), on the other hand, only produces a weak reaction with dilute acid if powdered. This is one way to distinguish the mineral dolomite from calcite, and the rock dolomite from limestone. [3] Dolomite rock is quite common (there’s a whole Italian mountain range named after it), which is surprising since marine organisms don’t make dolomite. All of the dolomite found in ancient rocks has been formed through the chemical process of magnesium replacing some of the calcium in the calcite in carbonate muds and sands. This process is known as dolomitization, and it is thought to take place where magnesium-rich water percolates through the sediments in carbonate tidal flat environments.
Chert

Not all marine organisms make their hard parts out of calcite; some, like radiolarians and diatoms, use silica (SiO2), and when they die their tiny shells (or tests) settle slowly to the bottom where they accumulate as chert. In some cases, chert is deposited along with limestone in the moderately deep ocean, but the two tend to remain separate, so chert beds within limestone are quite common (Figure 5.4.4), as are nodules, like the flint nodules within the Cretaceous chalk of southeastern England. In other situations, and especially in very deep water, chert accumulates on its own, commonly in thin beds.
Evaporites
In arid regions many lakes and inland seas have no stream outlet and the water that flows into them is removed only by evaporation. Under these conditions, the water becomes increasingly concentrated with dissolved salts, and eventually some of these salts reach saturation levels and start to crystallize (Figure 5.4.5). Although all evaporite deposits are unique because of differences in the chemistry of the water, in most cases minor amounts of carbonates start to precipitate when the solution is reduced to about 50% of its original volume. Gypsum (CaSO4·H2O) precipitates at about 20% of the original volume and halite (NaCl) precipitates at 10%. Other important evaporite minerals include sylvite (KCl) and borax (Na2B4O7·10H2O). Sylvite is mined at numerous locations across Saskatchewan from evaporites that were deposited during the Devonian (~385 Ma) when an inland sea occupied much of the region.

Coal
Coal, the first fossil fuel to be widely used, forms mostly on land in swampy areas adjacent to rivers and deltas in areas with humid tropical to temperate climates. The vigorous growth of vegetation leads to an abundance of organic matter that accumulates within stagnant water, and thus does not decay and oxidize. This situation, where the dead organic matter is submerged in oxygen-poor water, must be maintained for centuries to millennia in order for enough material to accumulate to form a thick layer (Figure 5.4.6a). At some point, the swamp deposit is covered with more sediment — typically because a river changes its course or sea level rises (Figure 5.4.6b). As more sediments are added, the organic matter starts to become compressed and heated. Low-grade lignite coal forms at depths between a few 100 m and 1,500 m and temperatures up to about 50°C (Figure 5.4.6c). At between 1,000 m to 5,000 m depth and temperatures up to 150°C m, bituminous coal forms (Figure 5.4.6d). At depths beyond 5,000 m and temperatures over 150°C, anthracite coal forms.
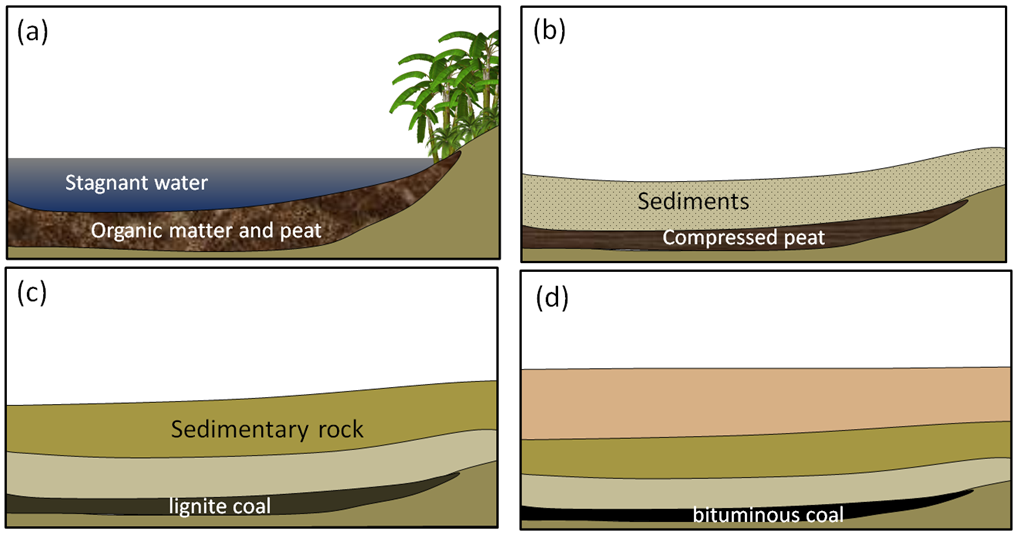
There are significant coal deposits in many parts of Canada, including the Maritimes, Ontario, Saskatchewan, Alberta, and British Columbia. In Alberta and Saskatchewan, much of the coal is used for electricity generation. Coal from the Highvale Mine, Canada’s largest, is used to feed the Sundance and Keephills power stations west of Edmonton. Almost all of the coal mined in British Columbia is exported for use in manufacturing steel.
Media Attributions
- Figures 5.4.1, 5.4.2, 5.4.3, 5.4.4, 5.4.5, 5.4.6: © Steven Earle. CC BY.
- Figure 5.4.2c: JoultersCayOoids by Wilson44691. Public domain.
- We use the word marine when referring to salt water (i.e., oceanic) environments, and the word aquatic when referring to freshwater environments. ↵
- Note that the term "fossiliferous" can also be used to modify the name of a clastic sedimentary rock (e.g., shale, mudstone, sandstone) that contains recognizable evidence of past life. ↵
- Dolomite is both a name for a mineral and for a rock composed of the mineral dolomite (although some geologists use the term dolostone to avoid confusion). ↵
a sedimentary rock that is comprised mostly of calcite
a microcrystalline chemical sedimentary rock formed almost entirely of silica
a type of soft white limestone formed from the skeletal remains of sea creatures
various different types of minerals and rocks that form when bodies of water evaporate
a rock composed of a single mineral
a rock composed of interlocking crystals
a rock containing ooids (spheroidal particles <2 mm in diameter composed of thin concentric layers of calcite or quartz precipitated from a solution around a nucleus)
the texture in a rock formed from the accumulation of fragmented remains of living organisms, most commonly shell fragments
a rock that contains the remains of living organisms (fossils) or evidence of past life (footprints, burrows)
the texture in a rock composed of very fine non-crystalline material created by organic or chemical activity
the zone on the ocean side of a reef
the zone of shallow water on the shore-side of a reef
Spheroidal particles <2 mm in diameter composed of thin concentric layers of calcite or quartz precipitated from a solution around a nucleus. Typically formed in warm, shallow water with strong currents.
a weak, poorly-cemented type of limestone consisting almost entirely of shells and shell fragments
a form of travertine that is especially porous as it forms around existing vegetative material
a deposit of calcium carbonate that forms at springs, hot springs or within limestone caves
a cone-shaped speleothem that is suspended from the roof of a cave
a cone-shaped speleothem that forms on the floor of a cave
a solutionally-formed feature within a limestone cave (e.g., a stalactite)
the addition of magnesium to limestone during which some or all of the calcium carbonate (calcite) is converted to dolomite
The purpose of a map is not limited to navigation. Maps are used in the geosciences to show data or information in a spatial context. Maps are used to convey information about where observations were recorded in the field, or to show a geologist’s interpretations of the materials exposed or deposited on the Earth’s surface. Geologists often use topographic maps as a base on which to display the geological information or data they wish to share. A topographic map is a graphic two-dimensional representation of the three-dimensional surface of the Earth. The features shown on a topographic map may be divided into three groups:
- Relief - hills, valleys, mountains, and plains
- Water features - lakes, ponds, rivers, canals, swamps, and streams
- Cultural features - roads, railways, buildings, and land boundaries
You were introduced to some important concepts about maps in the Google Earth Tutorial and Lab 1 Exercises. You learned that all maps are drawn to scale; that is, a designated distance on the map is equal to a corresponding real distance on the actual surface of the Earth. You also learned that locations on maps are specified using grid systems that might include the geographic or UTM systems. This lab will build upon those concepts to give you a deeper understanding of what elements make a map and what information you can convey in a map.
Map scale
Map scale refers to the fixed ratio between the distance you measure on a map (or in satellite imagery or air photos) and the actual distance on the ground, in the real world. You were introduced to the concept of scale at the beginning of the term, when you completed the Google Earth Tutorial and Lab 1 Exercises. In that tutorial, you used a scale bar to understand distances in Google Earth. Scale bars, also called graphic scale, are one way to represent scale on a map. Graphic scales are used to quickly determine the distance between two points on the map, by comparing the length you measure on the map with your ruler to the scale bar. In Figure 8.3.1 below, each of the long bars on the scale is 2 cm long and represents a distance of 1 km on the ground. Let’s say you measured the distance between two points on a map to be 5 cm. By comparing that length to the scale bar, you can see that 5 cm on the map represents 2.5 km on the ground. Note that the scale bar may be distorted by your screen if you are reading this on an e-reader, smartphone or computer depending on your display settings.

Another way to represent map scale is using a representative fraction (R.F.). The representative fraction is a ratio written in numbers on a map. Two of the common scales for topographic maps in Canada are 1:250,000 and 1:50,000. A R.F. of 1:250,000 means that 1 unit of measurement on the map represents 250,000 of the same unit of measurement on the ground. You can use any unit of measurement (centimetres, inches) as long as you are consistent! A map with a scale of 1:250,000 means that 1 cm on the map is equal to 250,000 cm on the ground, and that 1 inch on the map is equal to 250,000 inches on the ground.
Practice Exercise 8.2
What R.F. is shown by the scale bar drawn above? Assume that each of the long bars on the scale bar is 2 cm in length. Hint: you will have to do some unit conversions!
See Appendix 2 for Practice Exercise 8.2answers.
Determining the scale of an air photo or satellite image
In some cases, you may want to create a map using a base image rather than a base map. Base images could include air photos, captured by a low-flying aircraft along a set flight path, or satellite imagery like the images available in Google Earth Pro. While images can provide valuable details not captured on a topographic map, they typically lack scale. You can calculate the scale of your base image by comparing the length of a distinctive feature in the base image and a topographic map using a simple formula:
National Topographic System (NTS)
Standard topographic maps, published by the provincial and federal governments, are usually bounded and divided by parallels of latitude and meridians of longitude. In Canada, topographic maps are subdivided into numerous sections and subsections for the purpose of indexing all of the topographic maps of the nation in the National Topographic System (NTS). All topographic maps have a National Topographic Index Number that indicates the scale of the map and helps catalogue all the NTS maps of Canada. For example, the 1:1,000,000 NTS map sheet 82 covers most of southern Alberta, and is divided into indexed subsections as shown in Figure 8.3.2. The relationship between map scale and index number is outlined in table below.
Map index number | Map scale |
82 | 1 : 1,000,000 |
82 NE | 1 : 500,000 |
82 O | 1 : 250,000 |
82 O/1 | 1 : 50,000 |
You should always begin the study of a map by observing the title which gives clues to the location of the map in its wider setting. For your reference, the 1:50,000 scale topographic map that covers the city of Calgary is located on NTS map sheet 82 O/1.
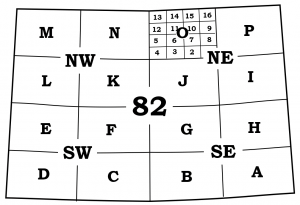
Three-dimensional relief on a two-dimensional map
Relief on a topographic map is illustrated using contour lines. These are imaginary lines drawn on a map to join points of equal elevation. Every point on a contour line is at the same elevation. Elevation is measured from a predetermined datum line: mean sea level, which is assigned an elevation of 0. Contour lines separate points of higher elevation (uphill) from points of lower elevation (downhill), therefore points between two contour lines are no lower than the contour line below and no higher than the contour line above. Contour lines never intersect or cross, except in very rare cases of overhanging cliffs. Contour lines always close; this closure may take place outside the map area as shown for the 50 m contour line in Figure 8.3.3. The contour interval (C.I) of a map is the difference in elevation between two adjacent topographic contour lines. The space between adjacent contour lines on a map reflects how steep or gentle the slope is: the closer the contour lines, the steeper the slope. Evenly spaced contour lines represent a uniform slope.
Practice Exercises 8.3
Examine the example topographic map in Figure 8.3.3. The map shows two hills separated by a small valley that has two streams.
- Which hill is the tallest? Or, in other words, which hill has the highest elevation?
- What is the approximate elevation of the red star?
- Toward which direction is each stream flowing?
- Can you see anywhere on the map where topography would be relatively steep?
- Can you see anywhere on the map where topography would be relatively gentle or flat?
- How can you tell the difference between areas with steep versus gentle topography using the contour lines?
- Notice that the 50 m and 100 m contour lines forms a “V” shape as they cross the stream. Topographic contour lines always form a “V” shape as they cross a stream, and the point of the “V” always points upstream. Imagine there is a third stream that originates at the red star and flows toward the NE. Draw in this third stream and using dashed lines, modify the contour lines where they cross this stream.
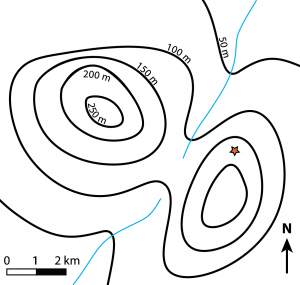
See Appendix 2 for Practice Exercise 8.3 answers.
Media Attributions
- Figures 8.3.1, 8.3.2, 8.3.3: © Siobhan McGoldrick. CC BY.