The Forestry Sector
Forestry has the most extensive footprint of all industrial sectors (Fig. 2.9) and it has been the primary target of environmental groups for many decades. As a result, sensitivity to concerns about biodiversity is relatively high, and broad commitments to ecological sustainability have been made.
Forestry sector commitments to ecological sustainability, combined with government downsizing, have resulted in a rather confused mandate for forestry companies. No longer are companies simply in the business of cutting down trees and selling wood products. Today, they are expected to serve as a land manager and achieve a broad range of social and environmental outcomes. Nevertheless, their authority over land use is limited, and the only outcome companies are actually paid for is the production of wood fibre.
A unique and important attribute of forestry companies is that they have the capacity to plan over ecologically meaningful spatial and temporal scales. A typical forest management area is thousands of square kilometres in size and planning extends over a full harvest cycle, implying a timeframe of over 100 years. Also, the registered forestry professionals employed by forestry companies bring a high standard of ecological literacy to the sector. Funding for the planning and implementation of conservation measures is provided mostly by forestry companies, as a cost of doing business, with secondary support from the government (e.g., for high-level planning, management oversight, and research).
There is also a strong institutional foundation for conservation within the forestry sector. There are enabling laws and conservation policies, along with provincial-level regulatory systems that promote sustainable forestry practices and mandate public input. Research centres within academia, government, and industry, along with industry associations, also help to support conservation.
Forestry-based conservation measures are implemented as part of a broader management framework referred to as sustainable forest management. The Canadian Council of Forest Ministers (2003) has developed a set of indicators that clarify the values that are to be sustained:
- Biological diversity
- The stability, resilience, and rates of biological production in forest ecosystems
- The quantity and quality of soil and water
- Global ecosystem functions, including the carbon cycle and hydrological cycles
- The flow of economic and social benefits from forests to current and future generations
- Decision making that is fair and effective and recognizes society’s responsibility to Indigenous people and forestry-dependent communities
The sustainable forest management framework has helped to raise awareness of non-timber values within the forestry sector and has led to a more balanced treatment of these values in forest management planning (Duinker 2011). It has also led to a tremendous amount of research into sustainable practices. Over the last three decades, our understanding of the ecology of forested systems has greatly advanced. Moreover, research has supported the development of new approaches to harvest planning and operating practices. A key advance has been the development of the natural disturbance model of forest harvesting.
The Natural Disturbance Model
Applied to the working landscape, the objective of maintaining ecological integrity embodies a fundamental contradiction. How can we hope to maintain a landscape that is natural while simultaneously allocating it for industrial development and other uses? This is the ecological equivalent of having our cake and eating it too.
The natural disturbance model (Landres et al. 1999) provides a partial solution based on the insight that disturbance itself is not the problem. Wildfire, storms, insect infestations, grazing, and other disturbances are a natural and necessary part of ecological systems (Fig. 7.3). Over evolutionary time, species have acquired the resilience needed to endure such disturbances without experiencing a long-term decline (Drapeau et al. 2016). Moreover, disturbance is needed to reset succession, generating the young and intermediate age classes that provide ecological niches for many species (Devictor and Robert 2009; Kuuluvainen and Grenfell 2012). The natural disturbance model posits that ecological integrity can be maintained in the face of active land use if human disturbances can be made to emulate natural disturbances (Long 2009; Drapeau et al. 2016).
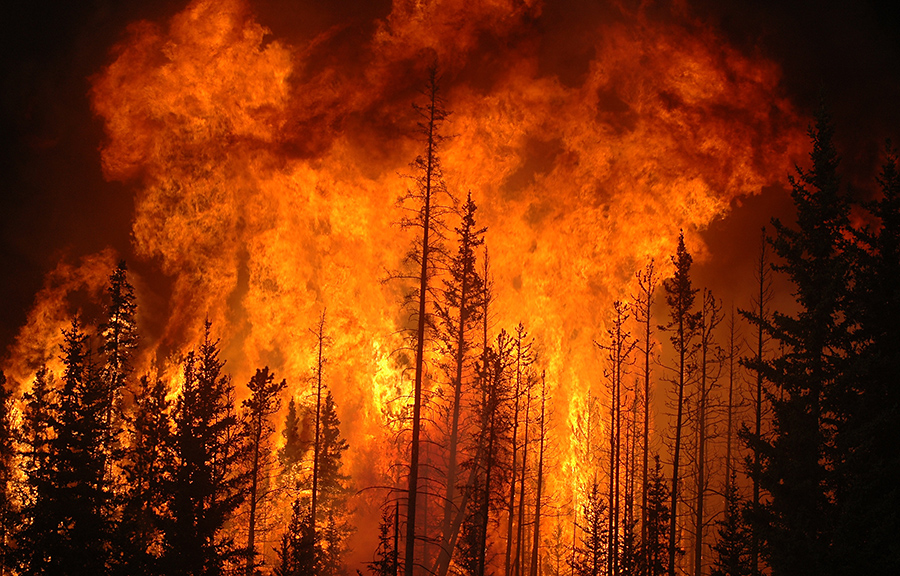
In practice, it is not the actual disturbance processes that are emulated, but the effects these disturbances have on ecosystem structures and patterns (Long 2009). Most applications have involved forested systems where wildfire, insect outbreaks, and windthrow are the dominant forms of natural disturbance (Kuuluvainen and Grenfell 2012). All of these types of disturbance can kill trees and reset successional trajectories, though they do not always do so. There is a great deal of variability in the intensity, location, timing, and extent of individual disturbance events, and this contributes to the complexity of forest ecosystems across multiple scales. Under the natural disturbance model, the aim is to understand this complexity and maintain it through appropriate harvesting practices (Shorohova et al. 2023).
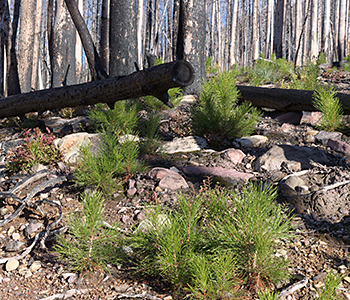
At the scale of individual forest stands, the initial consequence of stand-replacing disturbance is a dramatic change in stand structure. Most notably, the canopy is opened, allowing light and warmth to penetrate to the forest floor. Trees that have been killed often remain standing for many years, contributing structural complexity and a steady supply of dead wood to the forest floor (Fig. 7.4). Given the vagaries of the disturbance process, disturbed areas also tend to have remnant islands of living trees, which further contribute to local structural complexity (Perera et al. 2009).
Changes in stand structure engender changes in species composition (Swanson et al. 2011). In the absence of competition for sunlight and nutrients from mature trees, early successional vegetation is able to flourish. This in turn attracts animal species adapted to early successional habitats. There is also an increase in species specialized for using dead wood (Hannon and Drapeau 2005).
With time, stand composition and structure are remolded by successional processes (Fig. 7.5). The transition of stands to the mature stage is marked by closure of the canopy. Mature stands feature a dense growth of relatively even-aged trees and reduced understory development. The legacy of dead trees slowly diminishes.
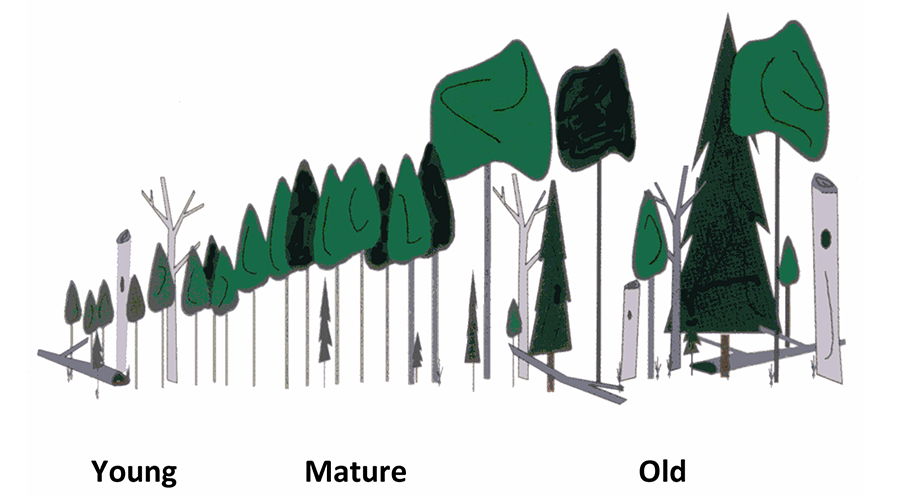
The transition from mature to old stands is gradual. The key changes include the appearance of canopy gaps from the death of individual trees, release of understory plants, emergence of secondary canopy species, and accumulation of snags and downed logs (Kneeshaw and Gauthier 2003). Relative to younger stages, old stands have trees of many ages and have more large canopy trees, large snags, and large downed logs. Stands with these characteristics are referred to as old-growth stands. The age at which this transition occurs varies among species because they mature at different rates. The high level of structural diversity in old-growth stands is associated with high species richness and the presence of many specialist species (Stelfox 1995; Martin et al. 2023).
At the landscape scale, the interplay between natural disturbances, regional climate, physical site conditions, and succession generates complex patterns (Yeboah et al. 2016). Forest patterns are best described as shifting mosaics because individual stands are in constant flux as a result of disturbance and succession (Fig. 7.6). Stability manifests at broader scales. For example, the distribution of stand ages across the entire forest may remain relatively constant, even as individual stands turnover. The same applies to the distribution of stand size and to measures of spatial configuration.
![]() |
Fig. 7.6. An aspen-spruce mixedwood forest illustrating the mosaic pattern that arises from the interplay of natural disturbance, site conditions, and local climate. Credit: R. Schneider. |
There are substantial differences in disturbance regimes among regions; therefore, forest patterns exhibit regional differences as well (Shorohova et al. 2023). The western boreal forest often experiences hot and dry conditions during the growing season, predisposing it to large fires (Johnson et al. 1998). Few stands escape burning long enough to reach the old-growth stage. For example, in northeastern Alberta, the mean interval between fires in any given location is approximately 70 years. Consequently, less than 20% of the forest is composed of old-growth stands (Larsen 1997; Al-Pac 2007). These old-growth stands exist as islands within a matrix of young forest.
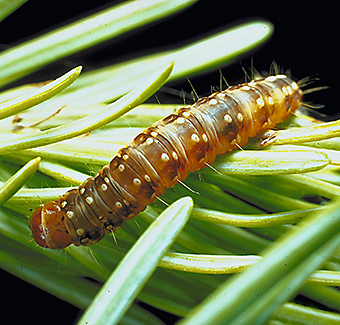
The rate of burning is lower in the eastern boreal forest because moisture levels are generally higher, and droughts are less frequent (Bouchard et al. 2008). For example, in eastern Quebec, the mean interval between fires is over 200 years. In the absence of harvesting, more than half of the forest is composed of old-growth stands (Belisle et al. 2011). Here it is young regenerating stands that are the islands, in a matrix of old forest. Over time, the old-forest matrix is sculpted by periodic insect infestations, which usually affect older trees (Fig. 7.7). In the eastern boreal forest, insects tend to modify stand structure through selective damage rather than causing stand-level mortality, though exceptions do occur (Bouchard and Pothier 2010).
Disturbance rates are lowest in the coastal rainforests of BC. Avalanches and windthrow are common sources of tree mortality here, though they usually do not affect large areas (Daniels and Gray 2006). Fires also occur but are very rare. Consequently, only 3% of the landscape is comprised of young and mature stands. The remaining 97% of the forest is in the old-growth stage (Pearson 2010).
Because of these regional differences in forest patterns and composition, the ecological reference state for each planning area must be determined using local data (Shorohova et al. 2023). Funding constraints and practicality limit the number of attributes and the level of detail that can be captured (Table 7.1). Priority is given to attributes relevant to guiding harvesting practices under the natural disturbance model (Patry et al. 2013). The attributes must be amenable to practical, reliable, and cost-effective measurement across large areas (Tear et al. 2005). This tends to exclude many of the functional aspects of ecological integrity, other than disturbance and succession. A working example is presented in Case Study 1.
Table 7.1. Forest attributes commonly used to characterize the ecological reference state under the natural disturbance model.1
Category | Attributes |
Stand composition | Stand area by type and age class2 |
Stand structure for early, mid, and late successional stages | Disturbance legacy (remaining live trees, standing dead trees, and snags) |
Amount of coarse woody debris on the forest floor | |
Presence of canopy gaps | |
Uniformity of tree ages within a stand | |
Landscape Patterns | Stand size distribution |
Stand shape and spatial arrangement, including the level of fragmentation | |
Spatial distribution of old‐growth stands and special features such as riparian zones | |
Ecological processes | Hydrologic function, including stream flow, turbidity, and connectivity3 |
Human disturbances with no natural analog | Roads, mines, well‐sites, hydroelectric dams, etc. |
1In larger study areas, the attributes shown here may be stratified by regional ecosystem type.
2Stand type is based on dominant vegetation and is used as a coarse‐filter proxy for overall stand composition.
3Disturbance and succession are captured through the stand structure and pattern attributes. Other ecological functions are not commonly measured because of practical constraints.
The ecological reference state is intended to represent the natural state of the system, so any legacies of past industrial use need to be excluded. The most practical way of reconstructing the natural state is through extrapolation from existing undisturbed areas, such as nearby parks, unallocated forest, and those parts of a forestry tenure that are excluded from harvest or are still awaiting their first pass. The main limitation of this approach is that the available undisturbed areas may not be representative of the planning area, either because they are too far away, too small for capturing landscape patterns, or unique in some way. An alternative approach is to use data from a period prior to substantial development, if it is available.
Another complication with reconstructing the ecological reference state from the current landscape is natural variability. As noted earlier, current forest patterns represent a snapshot out of a spectrum of possibilities. Forest age distribution, in particular, is very sensitive to the occurrence of large fires (Cumming et al. 1996). Large fires account for the bulk of the area burned over time, and their sporadic occurrence causes anomalies in the forest age distribution relative to the long-term mean.
Variability in fire occurrence can be handled by reconstructing the long-term, regional history of fires using fire records, tree-ring analysis, charcoal collection from lake sediments, and other techniques (Aakala et al. 2023). Adjustments need to be made to account for anthropogenic fires and the effects of fire suppression, which distort natural patterns. Using these techniques, fire history can often be traced for 200–300 years, permitting estimation of the long-term mean rate of burning and its temporal variance (Belisle et al. 2011). This information can then be used to roughly bound the NRV of the forest age distribution and to derive the natural proportion of old-growth forest.
Variability in stand structure and stand shape are easier to characterize. Most differences are due to fine-scale fire behaviour and subsequent successional processes (Andison and McCleary 2014). Local fire behaviour is in turn largely driven by topography and local weather and fuel conditions (Smyth et al. 2005). So we do not need to reach into the distant past to capture the range of possibilities (Andison and McCleary 2014). The current landscape provides a record of fires that burned under a wide variety of conditions and can provide a reasonable estimate of the NRV of most structural and shape attributes.
The Natural Disturbance Model in Practice
The natural disturbance model has been widely adopted as a tool for guiding forestry operations across Canada, evidenced by its incorporation in government planning manuals in many jurisdictions (Bergeron et al. 2007; Patry et al. 2013). The most notable operational changes that have been implemented include:
- A transition from fixed-size harvest blocks to harvest blocks of variable size
- A transition from square harvest blocks to blocks that emulate natural stand contours
- The on-site retention of snags and downed woody debris during harvest
- The retention of clumps of live trees within harvest blocks (Fig. 7.8)
- The regeneration of mixed species stands in areas where they naturally exist, instead of planting monocultures
- The retention of old-growth stands or old-growth characteristics on the landscape
- A reduction in salvage logging of burned forests
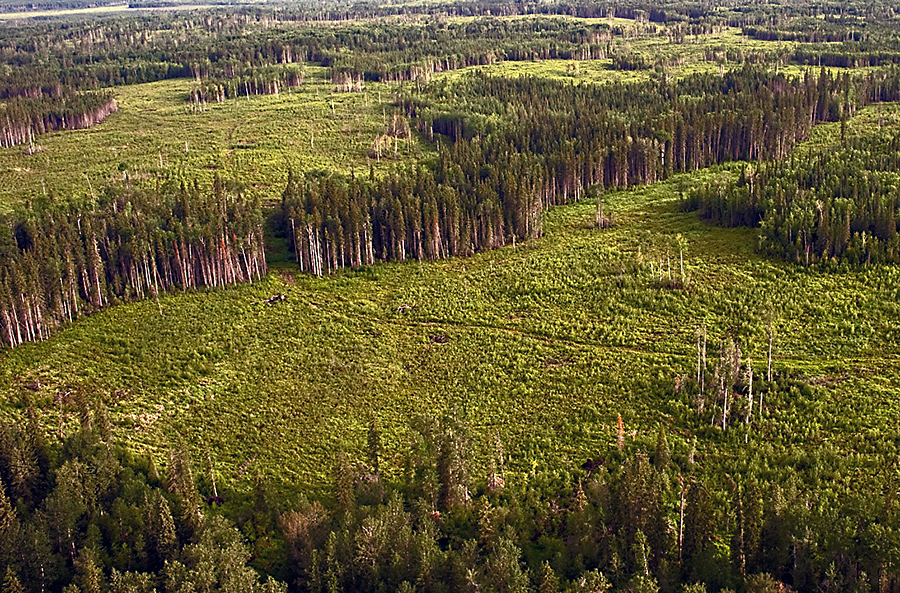
These changes represent a substantial improvement over the earlier system of sustained-yield management. However, conservation efforts remain constrained by a variety of factors (Gauthier et al. 2023). In particular, the calculation of annual harvest rates continues to be based on sustained-yield principles rather than the maintenance of ecological integrity (Burton et al. 2006).
Modelling studies (Armstrong et al. 1999), and experience from implementing the natural disturbance model in the US Pacific Northwest (MacCleery 2008), both indicate that maintaining forest integrity requires a substantial reduction in harvest intensity relative to sustained-yield practices. However, harvest intensity in Canada has only decreased marginally since the introduction of the natural disturbance model (Boyd 2003). With the addition of new forest tenures across the country, the overall area of forest harvested in Canada has actually increased relative to the sustained-yield era.
Additional barriers to implementing the natural disturbance model include:
- Uneven political support. Although all provinces have committed to sustainable forest management, through the Canadian Council of Forest Ministers, the level of support and implementation is uneven across the country (Gauthier et al. 2023).
- Lack of integration. Sustainable forest management is squarely focused on managing the effects of the forest industry. The ecological effects of other industrial operators in the same area are generally not considered.
- Absence of local advocates for biodiversity. Much of the decision making about conservation measures occurs at the local level, in the context of harvest planning. Although public input has been enhanced under sustainable forest management, most of this input comes from local communities which are dependent on the forest industry for employment. The biocentric views held by a broad segment of Canadian society are often underrepresented.
- Knowledge gaps. Despite all the research that has been undertaken in recent decades, the implementation of conservation measures is still constrained by knowledge gaps and uncertainty about the effects of potential management actions.
If we examine the implementation of the natural disturbance model at the level of individual forest attributes, we see that the level of implementation is a function of the associated socio-economic consequences (Drever et al. 2006). For attributes that can readily be emulated, like harvest block shape, managers often seek to maintain the attribute within NRV. But attributes that present trade-offs with other objectives often have management targets that fall significantly short of the conservation ideal (see Case Study 1).
The retention of old-growth forest is a case in point (Gauthier et al. 2023). Efforts to maintain natural amounts of old-growth on the landscape have a direct impact on timber supply and conflict with the conventional practice of truncating the forest age distribution at the optimal harvest age (see Fig. 5.9). Consequently, economic feasibility, rather than NRV, is usually the decisive factor in determining old-growth targets (Patry et al. 2013).
In the western boreal forest, where old-growth stands are relatively rare because of frequent fires, retaining natural amounts of old-growth is feasible as long as the timber supply has not been overallocated (Al-Pac 2007). However, forestry companies must be suitably motivated. In the eastern boreal region, where old-growth comprises a much larger proportion of the forest, natural amounts of old-growth cannot be retained without significant economic disruption. Here, a two-pronged strategy is being explored that uses floating old-growth reserves (see Chapter 8) to extend the age-class distribution of the forest, and silvicultural techniques to emulate old-growth features in younger managed stands (Belisle et al. 2011). Attempts are also being made to implement the triad approach (discussed below) as a way of enabling more old-growth retention while sustaining timber flows. Coastal forests, where disturbance rates are extremely low, present the most difficult case. In these long-lived forests, old-growth retention essentially means permanent protection. Indeed, protected area initiatives, many of them fractious, have been a defining feature of conservation efforts in this region.
Efforts to emulate fire skips (patches of unburned forest) through live tree retention also affect wood supply and are therefore subject to economic constraints. The same applies to maintaining representation of burned forest and insect-killed forest through restrictions on post-disturbance salvage logging. Consequently, management targets for these attributes are typically outside of NRV.
Constraints also exist for emulating the full size range of natural disturbances. In this case, the constraint is public opposition to large harvest blocks rather than economic concerns. When large harvest blocks are used, fewer access roads are needed, which is desirable from a conservation perspective (Carlson and Kurz 2007). But for a broad segment of the public, bigger is simply perceived as worse, reflecting a general aversion to forest harvesting. Moreover, many people mistrust industry and are reluctant to believe that the harvesting of large blocks will actually lead to less harvesting elsewhere. Instead, they perceive the use of large harvest blocks as a slippery slope toward an increased rate of harvest. Because of this opposition, harvest block size is generally capped in the 200–300 ha range, which is orders of magnitude lower than the openings created by large fires (OMNR 2009).
In summary, application of the natural disturbance model should, in principle, ensure that forest attributes remain within NRV despite forest harvesting. However, its implementation generally falls short of the ideal (Gauthier et al. 2023). Our ability to quantify complex forest structures and patterns is limited, as is our ability to emulate those attributes through harvesting. More importantly, the entire process is subject to socio-economic constraints. Consequently, some forest attributes are destined to shift outside of NRV as harvesting proceeds, to the detriment of biodiversity (Grondin et al. 2018).
Zonation
Managing for multiple objectives on the same piece of land unavoidably entails compromise, and as a result, it is often impossible to achieve all objectives satisfactorily. An alternative is to divide the land base into distinct zones that give priority to different management objectives (Anderson et al. 2012). A prime example is the establishment of formal protected areas to support biodiversity, which we will discuss separately in Chapter 8. Here we will examine other forms of zonation commonly used in the Industrial Forest to support conservation.
A form of zonation that has received widespread attention in the conservation literature is the “triad” approach (Hunter and Calhoun 1996; Cote et al. 2010). The triad approach adds an intensive management zone to the conventional dichotomy of protected areas and industrial-use areas. The concept was developed in a forestry context, where intensive management means plantations stocked with fast-growing tree species (often exotic or hybrid stock) and silvicultural practices that maximize growth. Additional economic gains are realized from locating the plantations near mills, which reduces road construction and hauling costs (Anderson et al. 2012).
The additional harvest volume generated on plantations is intended to offset production losses elsewhere on the landscape from new protected areas and the robust implementation of the natural disturbance model (Cote et al. 2010). By concentrating timber harvesting in a smaller area there is also a reduction in overall road density (Tittler et al. 2012).
Though the benefits of the triad approach have been demonstrated through modelling studies (Anderson et al. 2012; Tittler et al. 2012), there are few real-world examples. Attempts were made in BC and Alberta but were eventually abandoned because of changes in company ownership and changing economic circumstances (Tittler et al. 2016). The leading example at present is a project in Mauricie, Quebec, which is intended to serve as a pilot for future widespread application of the triad approach in Quebec (Messier et al. 2009).
The limited uptake of the triad approach can be attributed to institutional barriers and to uncertainties that reduce confidence in the expected outcomes. For conservationists, the concern is that, in the absence of legislation, the quid pro quo of increased protection may be less than promised or may not be maintained through time. Low-intensity management zones remain potential targets for future development, whereas plantations cannot easily be restored to natural forest. Furthermore, many conservationists reject the assumption that current harvest levels need to be maintained.
For forestry companies, the concern is that projected gains from intensive management may not be realized. Fire, insect outbreaks, and changing economic conditions may threaten the investments made in establishing plantations and may also lead to future shortages in timber supply (Ward and Erdle 2015). For governments, adopting the triad approach involves major changes in tenure and regulatory systems, which are not readily undertaken.
Another form of zonation involves the designation of special management zones (Nitschke and Innes 2004). In this approach, many different types of zones are possible, not just three. For example, special management zones have been used to provide extra protection of caribou calving grounds and to provide buffers around protected areas. They can also be applied to ecological features such as old-growth stands and riparian areas that are difficult to represent in protected areas because they are widely dispersed. In such cases, the sites are usually identified and managed by resource companies as a component of operational planning.
Most provinces employ some form of special management zoning, but there is little consistency in how they are designated or governed. Many special management zones are used to provide ad hoc protection to features of high conservation value where full protection is not feasible. In these cases, protective measures typically involve selective or seasonal prohibitions that are designed to achieve specific conservation objectives. The level of protection generally falls short of the blanket prohibitions of conventional protected areas but is greater than what is provided by generic regulations.
BC’s regional land-use planning initiatives of the 1990s and 2000s made extensive use of zonation as a planning tool (Fig. 7.9; FPB 2008). For each planning region, multiple zones and associated management priorities were designated by government planning teams working in collaboration with stakeholders. Zonation was used as a way of proactively tackling land-use conflicts that remained hidden and unresolved under conventional multiple-use management. The number and types of zones varied from region to region, reflecting regional geophysical and ecological differences as well as differences in the priorities of local stakeholders (Nitschke, 2008).
![]() |
Fig. 7.9. An illustration of zonation, as applied in the Kamloops Land and Resource Management Plan, in southeast BC. SMZ = Special Management Zone. Adapted from KIMC 1995. |
![]() |