Species‐Level Conservation
Recovery Plans
Species recovery plans have three main components: species information, a set of management objectives, and proposed management actions. Adjustments to each of these components are needed to accommodate climate change.
The species information component should include a formal vulnerability assessment that examines climate exposure as well as sensitivity. This assessment should take both short-term changes (i.e., increased climate variability) and long-term changes (i.e., progressive warming) into account. The potentially positive effects of climate change should also be considered.
Once the preindustrial baseline fades from relevance, a dynamic reference state will need to be used when setting recovery objectives. The procedures for doing so have not yet been worked out, but presumably will entail some form of habitat and population modelling. The concern is mainly with long-term recovery objectives that reference the natural state. This state is now a moving target.
Short-term recovery objectives do not require much adjustment because they focus on population survival and reversing population declines. The relevant reference points, such as minimum viable population size, are determined largely by proximate risk factors.
Finally, recovery plans should include species-specific conservation measures for mitigating climate-related threats and facilitating adaptation.
In the near term, the greatest concern for most species is increased climatic variability and extreme weather events (Parmesan et al. 2000). This issue should be addressed like other conventional threats, through direct mitigation measures. For example, it is expected that an increase in extreme rainfall events will cause more acute food shortages for grassland raptors (Fisher et al. 2015b). This can be mitigated through habitat management that increases the abundance and availability of prey, and when necessary, through supplemental feeding.
The long-range threats posed by climate change are more challenging to address because the amount and timing of the changes are uncertain. There is also less certainty about how effective our actions are likely to be. Finally, it is often difficult to obtain adequate funding because long-term risks may be perceived as less important than immediate threats. In this respect, climate change is similar to other “slow-creep” issues, such as industrial cumulative effects, which have proven difficult to address.
The most effective approach for helping species cope with progressive warming is to increase their resilience and adaptive capacity (Seidl 2014). This entails reducing the current causes of stress, whatever they may be, using conventional conservation measures. It also includes reducing barriers to movement. While not novel, these are essential components of adaptation and should not be overlooked.
Another approach is to slow the rate of ecological transitions to buy time for species to adapt. This runs counter to the concept of facilitating natural transitions, but it may be needed as a temporary measure to help species that are unable to keep pace with rapid warming. For example, we might try to reduce the rate of anthropogenic and natural disturbances (like fire) that mediate ecological transitions. Or we might seek to slow the influx of disruptive species from warmer regions. Forestry replanting programs can help slow transitions in forested landscapes.
Lastly, there are mitigation measures that are part of the conventional conservation toolkit but are used differently under climate change. The two main methods that fall into this category are protected areas and assisted migration.
Protected Areas
The use of protected areas for conserving focal species is far more difficult under climate change because their preferred habitat becomes a moving target (Sandler 2013). Two approaches are available and both have major shortcomings.
The first approach is a “floating” reserve system in which protected areas shift location in synch with changes in the climate envelope of the species of interest. The main drawback of this approach is that it forces us to trade relatively pristine landscapes inside existing reserves for degraded landscapes elsewhere, defeating the core purpose of protection (Rayfield et al. 2008). Furthermore, the logistics of implementing a floating reserve system at broad scales for multiple focal species seem insurmountable.
The second approach is to represent both the current and future habitat needs of focal species within a fixed reserve system (Schuetz et al. 2015). This approach is conceptually sound but impractical when the amount of land available for protection is constrained, as it usually is. With even a small number of focal species, a requirement to represent all future contingencies could easily entail protecting most of the land base. Another shortcoming of this approach is that it is heavily dependent on long-range projections of bioclimatic envelope models, which are subject to considerable uncertainty, particularly after 2050.
Because of these limitations, focal species representation should be deemphasized in systematic conservation planning initiatives, in favour of the more robust coarse-filter approach. The dilemma for conservation practitioners is that focal species are highly valued by the public and stakeholders and cannot simply be ignored. A solution is to include focal species in the planning process, but mainly for informational purposes. The intent is to determine how well an optimally configured coarse-filter design will protect selected focal species, both now and into the future. If significant deficiencies are noted, then the costs and benefits of alternative designs can be investigated and discussed (preferably in a structured decision-making framework). The high uncertainty of climate envelope projections should be formally included in these deliberations.
Assisted Migration
In contrast to the other conservation measures we have examined, assisted migration (or assisted colonization) entails direct intervention in the adaptation process. It is also one of the more controversial measures and the subject of ongoing debate (Ricciardi and Simberloff 2009; Hewitt et al. 2011). The objective is to help species keep pace with climatic changes by physically moving them to new locations. As such, it can be viewed as an extension of the reintroduction methods we discussed in Chapter 6.
To date, assisted migration has been applied mainly in the forestry sector, where the focus is on maintaining forest productivity. BC is leading the way in Canada, with its development of a new climate-based seed transfer system (O’Neill et al. 2017). Seed transfer systems provide the rules forestry companies must follow when sourcing seed stock for their reforestation programs. These rules ensure that the seed stock is matched to the local climate, which in the past has meant limiting seed transfers to their zone of origin. To facilitate adaptation to climate change, BC’s new system allows companies to use stock from zones that are slightly warmer. Other provinces are contemplating similar changes but are at an earlier stage of implementation (Klenk 2015).
Because BC’s new seed transfer system involves moving populations within the existing range of a species, there is little concern about invasiveness. Moreover, rather than targeting some distant point in the future, the system is designed to match seed stock to the climate conditions 12–17 years from the present (O’Neill et al. 2017). The intent is mainly to help the selected species “catch up” with the climate shifts that have already occurred. As a result, the implementation of this system has not been very controversial (Klenk 2015).
It is possible that more aggressive assisted-migration programs will be implemented by the forestry sector in the future. The field is evolving rapidly, and considerable research is underway, including growth trials of non-native species (Thorpe et al. 2006). The inherent risks have yet to be fully assessed, and it is possible that implementation efforts will at some point face public resistance.
Assisted migration is also being explored as a conservation measure for maintaining biodiversity (Gallagher et al. 2015; Vitt et al. 2016). There are several situations where assisted migration might be used. One involves species that are unable to keep pace with moderate to fast rates of climate change because their capacity for migration is low. If conditions along the trailing edge of their range become clearly unsuitable, leading to population declines, it would be better to move the affected populations to a more viable area rather than simply allowing them to become extirpated.
Another situation involves species that are contracting to the core of their range because of anthropogenic stress (recall the burrowing owl example). These species are unlikely to keep pace with changes in their climate envelope without human intervention.
Finally, assisted migration may be warranted for species that are unable to shift their range effectively because of an anthropogenic barrier. Species at risk in southern Canada are a prime example. Many of these species may require assistance in shifting their range northward because of the barrier presented by agricultural lands.
The use of assisted migration for biodiversity applications is still at an early stage, and there are a variety of challenges to be overcome (Hancock and Gallagher 2014; Gallagher et al. 2015). The issue that has received the most attention, and has been the main cause of controversy, is the potential for the transplanted species to disrupt the recipient ecosystem. Species transfers have a checkered history, often involving unintended consequences. For example, the red squirrel was introduced to Newfoundland in 1963 in an attempt to bolster the island’s struggling American marten population (Benkman 1993). Unfortunately, the introduction of the squirrels, which are not native to Newfoundland, led to increased competition for conifer cones which hastened the decline of Newfoundland’s threatened red crossbill (COSEWIC 2016).
Proponents of assisted migration maintain that climate adaptation applications are not comparable to past species introductions and carry much less risk (Schlaepfer et al. 2009; Vitt et al. 2009). Assisted migration is expensive and time-consuming, so it is unlikely to be used for species that can shift their range without assistance. This eliminates species with invasive potential. Furthermore, the target habitats lie along climate gradients that the species could and would reach naturally if not for anthropogenic barriers and ecological stressors. Finally, the intent is not to place species into new ecosystems, but to help them maintain their position in existing ecosystems as they shift location in response to climate change.
The other factors to be considered are common to all species reintroduction programs (see Chapter 6). These include knowledge about the ecology of the species, knowledge about effective translocation methods, the availability and viability of founder populations, the availability of suitable transplant locations, current and future threats to population viability, and stakeholder and institutional support (Gallagher et al. 2015). Cost is an overriding factor and will likely limit the widespread application of assisted migration, just as it limits reintroduction and restoration projects today.
In summary, both the promise and risks of assisted migration are less than they might appear. Given capacity constraints and ecological limitations, the reality is that most species in most areas will have to adapt to climate change on their own. Nevertheless, assisted migration is a conservation tool that is worth exploring as part of the strategic planning process for species at risk. As always, the question for managers is how the costs and benefits compare to other available management options (Schlaepfer et al. 2009). Pilot studies are a logical first step, providing the information needed to make informed decisions about broader applications (Fig. 9.15).
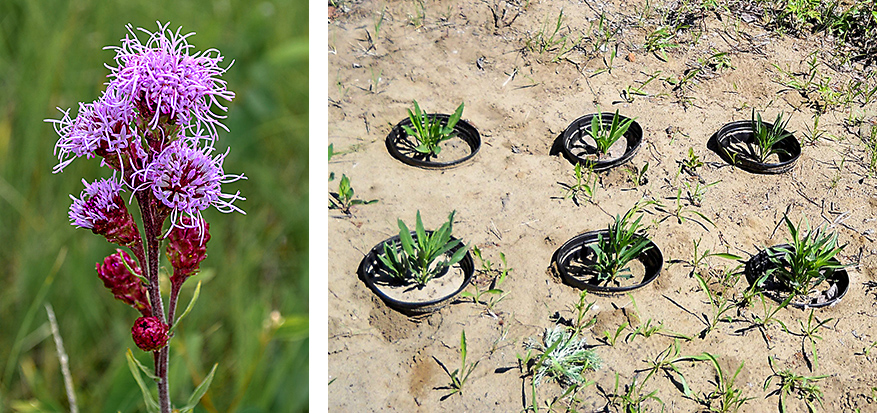
Population-Level Triage
In Chapter 6, we discussed the concept of conservation triage in the context of prioritizing species. Triage has also been proposed at the population level, as a response to climate change (Lawler 2009; Hagerman and Satterfield 2014). The basic argument is that, for many species, populations along the trailing edge of the range are unlikely to persist under warmer temperatures. Therefore, to obtain the greatest benefit from limited conservation resources, we should redirect conservation resources from trailing-edge populations to leading-edge populations.
The conservation of salmon along the Pacific coast of Canada and the US provides a case in point. Hundreds of millions of dollars are spent annually on the conservation of salmon up and down the coast (Barnas et al. 2015). Because of climate change, the maximum summer water temperature in many southern streams is now approaching and occasionally exceeding the thermal limits of salmonids (Honea et al. 2016). As temperatures continue to increase over the course of this century, southern salmon populations are expected to decline, despite restoration efforts (Fig. 9.9). Coincidently, various salmon species are now being observed in Arctic waters, indicating that losses of southern populations are likely to be offset by gains in northern areas (Nielsen et al. 2013). From this broader perspective, a triage approach that redirects conservation resources from southern to northern populations seems warranted.
While these arguments are compelling, a major shortcoming is that they frame the decision too narrowly. As we saw in Chapter 6, triage solutions will not be broadly optimal if important dimensions of the problem are omitted. In this case, attention is narrowly focused on the local persistence of individual populations while other relevant factors, such as genetic adaptations and range shifts, are not considered.
If we abandon warm-adapted trailing-edge populations, allowing them to become quickly extirpated, we stand to lose part of the gene pool (Savolainen et al. 2007; Martins et al. 2012). As a result, the species may experience a permanent contraction in range because it can no longer accommodate the same spectrum of environmental conditions (Fig. 9.16; Hampe and Petit 2005). Thus, trailing-edge populations still merit conservation, even though they may not persist in their current locations (Hampe and Petit 2005; Battin et al. 2007). The objective is to maintain these populations long enough for gene flow to occur into northern regions.
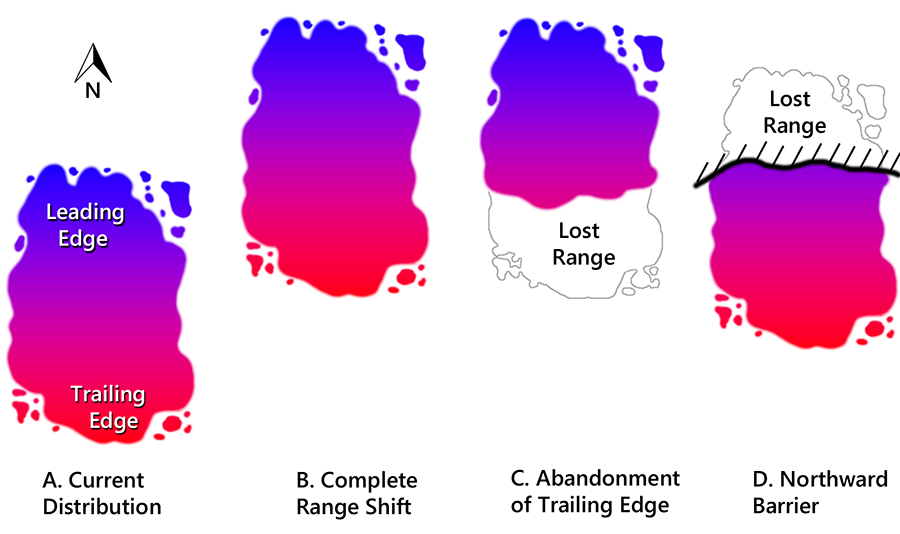
Another consideration is the practicality of the funding reallocations that are central to the triage approach. Even if population-level triage provides a clear conservation benefit, this does not mean local authorities will necessarily agree to transfer funding to distant jurisdictions over which they have no control or responsibility. The political optics of formally abandoning a local population are also highly problematic for regional governments.
These shortcomings do not mean triage is without merit. As conservation practitioners, we should always seek to optimize the allocation of limited conservation resources (Bottrill et al. 2008). And we must accept that this will sometimes involve unpalatable choices. However, it is important to consider all relevant dimensions of a management problem. Furthermore, the progressive nature of climate change demands a dynamic framework for management—the transitions are just as important as the endpoints. Finally, the practical reality is that optimal resource allocation will always be easier to achieve at the local level, where organizations are allocating resources within their own domain. For issues that cross jurisdictions, we usually have to settle for coordinated planning rather than fully integrated planning.