6.1 Metamorphism and Plate Tectonics
Metamorphism is the change that takes place within a body of rock as a result of it being subjected to conditions that are different from those in which it formed. In most cases—but not all—this involves the rock being deeply buried beneath other rocks, where it is subjected to higher temperatures and pressures than those under which it formed. Metamorphic rocks typically have different mineral assemblages and different textures from their parent rocks, or protoliths, but they may have the same overall chemical composition.
Most metamorphism results from the burial of igneous, sedimentary, or pre-existing metamorphic rocks to the point where they experience different pressures and temperatures than those at which they formed. Metamorphism can also take place if cold rock near the surface is intruded and heated by a hot igneous body. Although most metamorphism involves temperatures above 150°C, some metamorphism takes place at temperatures lower than those at which the parent rock formed.
The main factors that control metamorphic processes are:
- the mineral composition of the protolith,
- the temperature at which metamorphism takes place,
- the amount and type of pressure during metamorphism,
- the types of fluids (mostly water) that are present during metamorphism, and
- the amount of time available for metamorphism.
Protolith
The protolith, or “parent rock”, is the rock that exists before metamorphism starts. Sedimentary or igneous rocks can be considered the parent rocks for metamorphic rocks. Although an existing metamorphic rock can be further metamorphosed or re-metamorphosed, metamorphic rock doesn’t normally qualify as a “parent rock”. For example, if a mudstone is metamorphosed to slate and then buried deeper where it is metamorphosed to gneiss, the parent rock of the gneiss is mudstone, not slate. The critical feature of the parent rock is its mineral composition because it is the stability of minerals that counts when metamorphism takes place. In other words, when a rock is subjected to increased temperatures, certain minerals may become unstable and start to recrystallize into new minerals, while remaining in a solid state.
Temperature
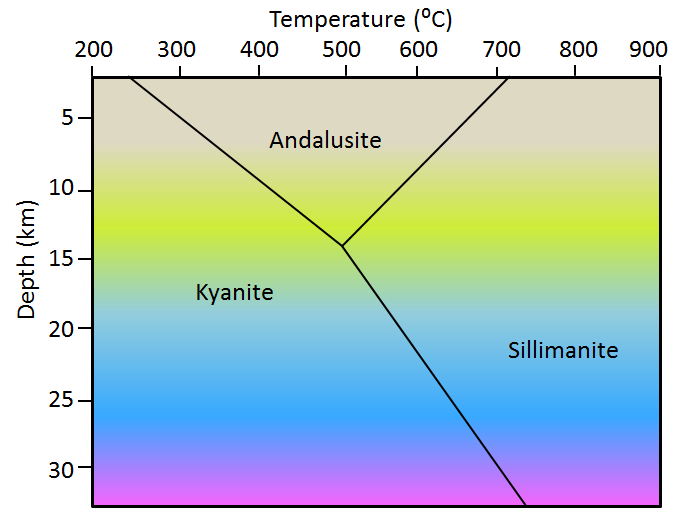
The temperature that the rock is subjected to is a key variable in controlling the type of metamorphism that takes place. As we learned in the context of igneous rocks, mineral stability is a function of temperature, pressure, and the presence of fluids (especially water). All minerals are stable over a specific range of temperatures. For example, quartz is stable from environmental temperatures (whatever the weather can throw at it) all the way up to about 1800°C. If the pressure is higher, that upper limit will be even higher. If there is water present, it will be lower. On the other hand, most clay minerals are only stable up to about 150° or 200°C; above that, they transform into micas. Most feldspars are stable up to between 1000°C and 1200°C. Most other common minerals have upper limits between 150°C and 1000°C.
Some minerals will crystallize into different polymorphs (same composition, but different crystalline structure) depending on the temperature and pressure. The minerals kyanite, andalusite, and sillimanite are polymorphs with the composition Al2SiO5. They are stable at different pressures and temperatures, and, as we will see later, they are important indicators of the pressures and temperatures that existed during the formation of metamorphic rocks (Figure 6.1.1).
Pressure
Pressure is important in metamorphic processes for two main reasons. First, it has implications for mineral stability (Figure 6.1.1). Second, it has implications for the texture of metamorphic rocks. Rocks that are subjected to very high confining pressures are typically denser than others because the mineral grains are squeezed together (Figure 6.1.2a), and also because they may contain minerals that have greater density because the atoms are more closely packed.
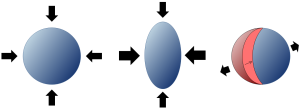
Because of plate tectonics, pressures within the crust are typically not applied equally in all directions. In areas of plate convergence, for example, the pressure in one direction (perpendicular to the direction of convergence) is typically greater than in the other directions (Figure 6.1.2b). In situations where different blocks of the crust are being pushed in different directions, the rocks will likely be subjected to shear stress (Figure 6.1.2c).
One of the results of directed pressure and shear stress is that rocks become foliated—meaning that they’ll develop a foliation or directional fabric. Foliation is a very important aspect of metamorphic rocks, and is described in more detail later in this chapter.
Fluids
Water is the main fluid present within rocks of the crust, and the only one that we’ll consider here. The presence of water is important for two main reasons. First, water facilitates the transfer of ions between minerals and within minerals, and therefore increases the rates at which metamorphic reactions take place. So, while the water doesn’t necessarily change the outcome of a metamorphic process, it speeds the process up so metamorphism might take place over a shorter time period, or metamorphic processes that might not otherwise have had time to be completed are completed.
Secondly, water, especially hot water, can have elevated concentrations of dissolved elements (ions), and therefore it is an important medium for moving certain elements around within the crust. So not only does water facilitate metamorphic reactions on a grain-to-grain basis, it also allows for the transportation of elements from one place to another. This is very important in hydrothermal processes, and in the formation of mineral deposits.
Time
Most metamorphic reactions take place at very slow rates. For example, the growth of new minerals within a rock during metamorphism has been estimated to be about 1 millimetre per million years. For this reason, it is very difficult to study metamorphic processes in a lab.
While the rate of metamorphism is slow, the tectonic processes that lead to metamorphism are also very slow, so in most cases, the chance for metamorphic reactions to be completed is high. For example, one important metamorphic setting is many kilometres deep within the roots of mountain ranges. A mountain range takes tens of millions of years to form, and tens of millions of years more to be eroded to the extent that we can see the rocks that were metamorphosed deep beneath it.
Practice Exercise 6.1
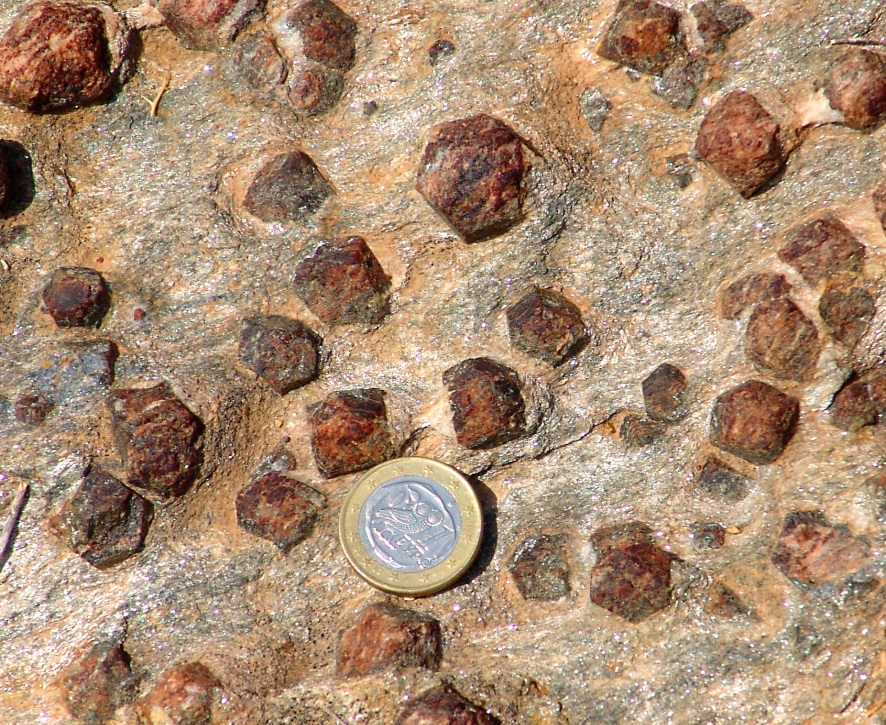
This photo shows a sample of garnet-mica schist from the Greek island of Syros. The large reddish crystals are garnet, and the surrounding light coloured rock is dominated by muscovite mica. The Euro coin is 23 millimetres in diameter. Assume that the diameters of the garnets increased at a rate of 1 millimetre per million years.
Based on the approximate average diameter of the garnets visible, estimate how long this metamorphic process might have taken.
Plate Tectonics and Metamorphism
All of the important processes of metamorphism can be understood in the context of geological processes related to plate tectonics. The relationships between plate tectonics and metamorphism are summarized in Figure 6.1.4. Two settings, continent-continent collisions and continental volcanic arcs are also shown in more detail in Figure 6.1.5.
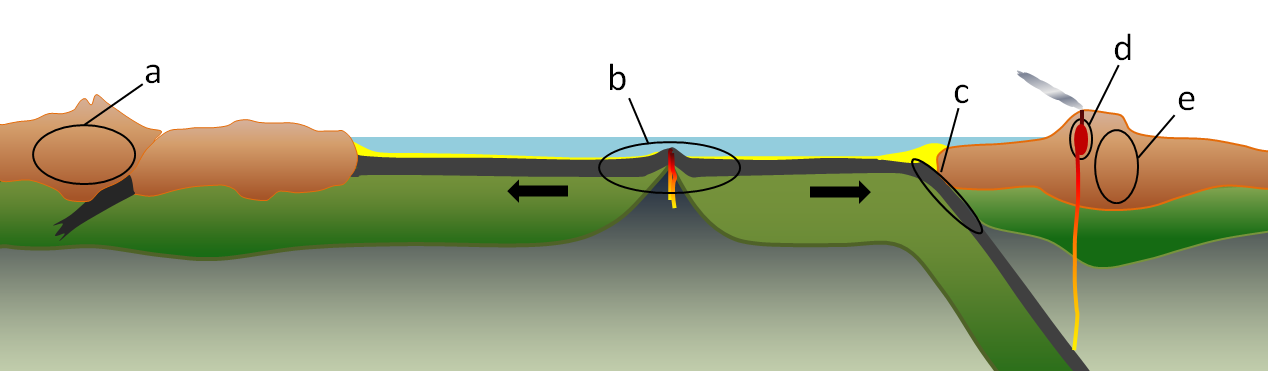
Most regional metamorphism takes place within the continental crust. While rocks can be metamorphosed at depth in most areas, the potential for metamorphism is greatest in the roots of mountain ranges where there is a strong likelihood for burial of relatively young sedimentary rock to great depths, as depicted in Figure 6.1.5. An example would be the Himalayan Range. At this continent-continent convergent boundary, sedimentary rocks have been both thrust up to great heights (nearly 9,000 metres above sea level) and also buried to great depths. Considering that the normal geothermal gradient (the rate of increase in temperature with depth) is around 30°C per kilometre, rock buried to 9 kilometres below sea level in this situation could be close to 18 kilometres below the surface of the ground, and it is reasonable to expect temperatures up to 500°C. Metamorphic rocks formed there are likely to be foliated because of the strong directional pressure (compression) of converging plates.
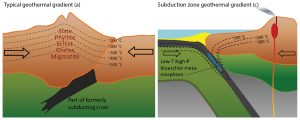
Metamorphism also occurs at subduction zones, where oceanic crust is forced down into the hot mantle. Because the oceanic crust is typically relatively cool by the time it reaches the subduction zone, especially along its sea-floor upper surface, it does not heat up quickly, and the subducting rock remains several hundreds of degrees cooler than the surrounding mantle (Figure 6.1.5 right). A special type of metamorphism takes place under these very high-pressure but relatively low-temperature conditions, producing an amphibole mineral known as glaucophane (Na2(Mg3Al2)Si8O22(OH)2), which is blue in colour, and is an important component of a rock known as blueschist.
You’ve probably never seen or even heard of blueschist; that’s not surprising. What is a little surprising is that anyone has seen it! Most blueschist forms in subduction zones, continues to be subducted, turns into eclogite at about 35 kilometres depth, and then eventually sinks deep into the mantle—never to be seen again because that rock will eventually melt. In only a few places in the world, where the subduction process has been interrupted by some other tectonic process, has partially subducted blueschist rock returned to the surface. One such place is near San Francisco in an area known as the Franciscan Complex.
Another way to understand metamorphism is by using a diagram that shows temperature on one axis and depth—which is equivalent to pressure—on the other (Figure 6.1.6). The three heavy dotted lines on this diagram represent Earth’s geothermal gradients under different conditions. In most areas, the rate of increase in temperature with depth is 30°C per kilometre. In other words, if you go 1,000 metres down into a mine, the temperature will be roughly 30°C warmer than the average temperature at the surface. In most parts of southern Canada, the average surface temperature is about 10°C, so at a 1,000 metre depth, it will be about 40°C. That’s uncomfortably hot, so deep mines must have effective ventilation systems. This typical geothermal gradient is shown by the green dotted line in Figure 6.1.6. At a 10 kilometre depth, the temperature is about 300°C and at 20 kilometres it’s about 600°C.
In volcanic areas, the geothermal gradient is more like 40° to 50°C per kilometre, so the temperature at a 10 kilometre depth is in the 400° to 500°C range. Along subduction zones, as described above, the cold oceanic crust keeps temperatures low, so the gradient is typically less than 10°C per kilometre. The various types of metamorphism described above are represented in Figure 6.1.6 with the same letters (a through e) used in Figures 6.1.4 and 6.1.5.
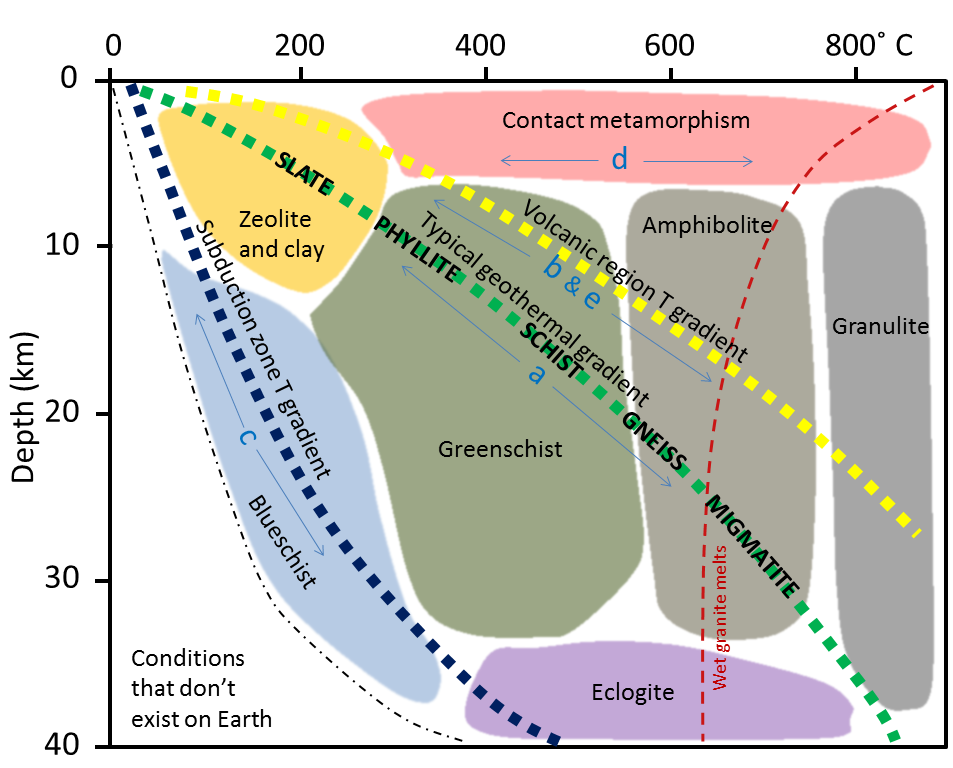
By way of example, if we look at regional metamorphism in areas with typical geothermal gradients, we can see that burial in the 5 kilometre to 10 kilometre range puts us in the clay mineral zone (see Figure 6.1.6), which is equivalent to the formation of slate. At 10 to 15 kilometres, we are in the greenschist zone (where chlorite would form in mafic volcanic rock) and very fine micas form in mudrock, to produce phyllite. At 15 to 20 kilometres, larger micas form to produce schist, and at 20 to 25 kilometres amphibole, feldspar, and quartz form to produce gneiss. Beyond a depth of 25 kilometres in this setting, we cross the partial melting line for granite (or gneiss) with water present, and so we can expect migmatite to form.
Practice Exercise 6.2 Metamorphic rocks in areas with higher geothermal gradients
Figure 6.1.6 shows the types of rock that might form from a mudrock protolith at various points along the curve of the “typical” geothermal gradient (dotted green line). Looking at the geothermal gradient for volcanic regions (dotted yellow line in Figure 6.1.6), estimate the depths at which you would expect to find the same types of rock forming from a mudrock protolith.
- Slate
- Phyllite
- Schist
- Gneiss
- Migmatite
Media Attributions
- Figures 6.1.1, 6.1.2, 6.1.4, 6.1.5, 6.1.6: © Steven Earle. CC-BY-4.0.
- Figure 6.1.3: Garnet Mica Schist Syros Greece © Graeme Churchard. CC-BY-2.0.