19 The Rock Cycle II: Sediment and Sedimentary Rock
Sedimentary processes
Next we are going to look at the part of the rock cycle that involves the atmosphere, hydrosphere, and biosphere. All of those external parts of the Earth act on the Geosphere, principally the crust, and change it. They produce material that is able to be moved around on the surface of the Geosphere. When that material is finally deposited on the surface of the Geosphere, it makes sediment and, eventually, sedimentary rock.
We will first look at the processes – weathering and erosion — that make sediment, and the processes that move it over the surface of the Geosphere. Some of these processes are covered in more detail in other sections, particularly those dealing with the Atmosphere and Hydrosphere. Then we will look at the deposition of the major categories of sediment and sedimentary rock. Once again, this involves processes in all the other “spheres” of the Earth: the Atmosphere, the Hydrosphere, and the Biosphere.
Learning Outcomes
- Distinguish the main processes of weathering and erosion that contribute sediment to the Earth’s surface
- Describe the main types of clastic and non-clastic sediment and sedimentary rock
- Identify the processes of diagenesis that convert sediment into sedimentary rock
Weathering and erosion
The processes by which the Biosphere, Atmosphere, and Hydrosphere modify the Geosphere are called weathering. We can recognize two big categories of weathering: physical weathering (also known as mechanical weathering), and chemical weathering.
Sometimes, products of weathering remain on the surface of the Geosphere more or less where they are formed. Such materials are known as regolith or soil. Often, however, material is removed from the site of weathering. When this happens we call the process erosion. Eroded material is typically transported over the surface of the Geosphere and deposited elsewhere as sediment.
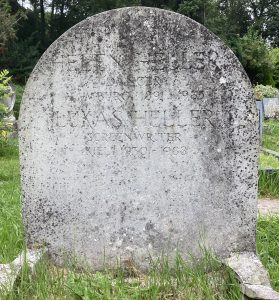
We can see the effects of weathering when we look at rocks that have been placed by humans in buildings and gravestones, which often bear a date, allowing us to judge the rate of weathering and erosion.
Physical weathering
Let’s look first at processes of physical weathering. Mechanical weathering does not involve major chemical change to the minerals in the rock. It just breaks up the rocks into smaller particles.
Frost action
One of the most important process in temperate and polar latitudes is [pb_glossary id=”3213″]frost action[/pb_glossary], described in the section on water. Frost action occurs when water penetrates into cracks in the rock. Those cracks can be very small. For example, tiny cracks occur between the minerals in a granite, which had are wide enough for a few molecules of water. From 4°C down to a temperature of about -20°C, water and ice expand by about 9% as they get colder. This expansion can exert very large forces on rocks, and it helps to break rocks apart wherever freezing and thawing take place, a process known as frost wedging. Frost action is most effective in climates where freezing and thawing occur many times in the year, and beneath wet-based glaciers.
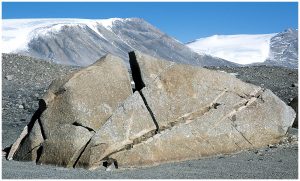
Abrasion
Abrasion refers to the breaking and grinding away of solid rock by collisions with moving particles. Abrasion takes place in many environments: fast-moving streams, shorelines subject to storm waves, desert environments with high winds and beneath glaciers that are loaded with fragments of rock.
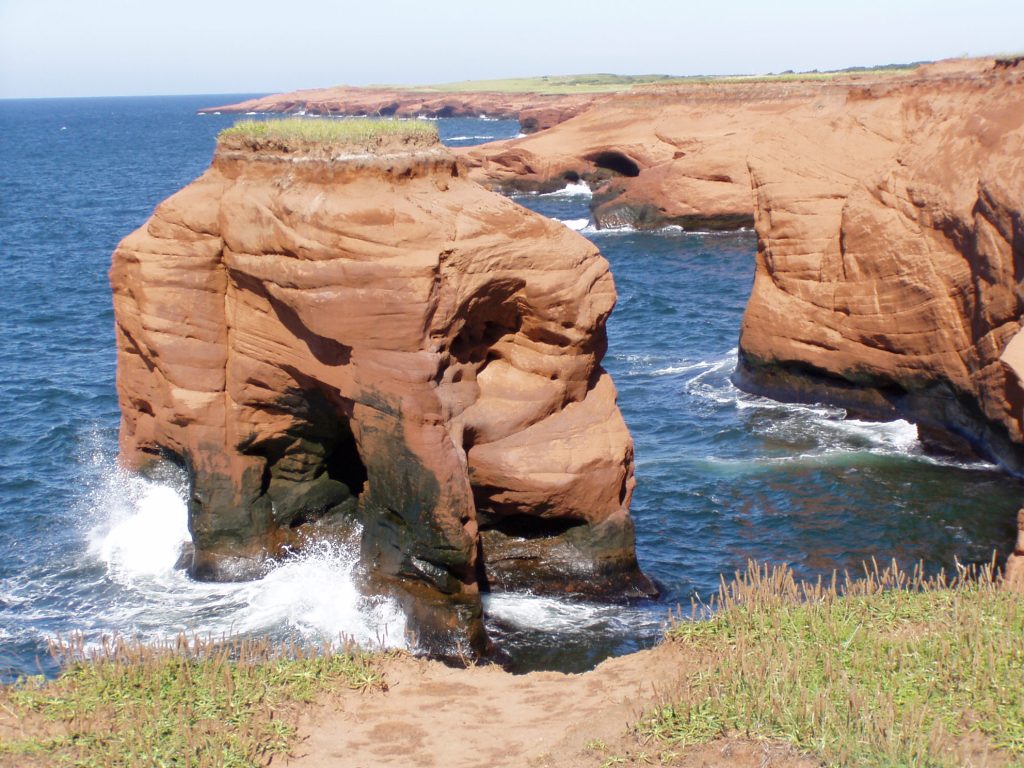
Pressure release
As erosion of the landscape takes place, rocks that were formerly deeply buried are brought closer to the surface. As they are brought closer to the surface, pressures are reduced. The slight expansion that results can cause mechanical weathering.
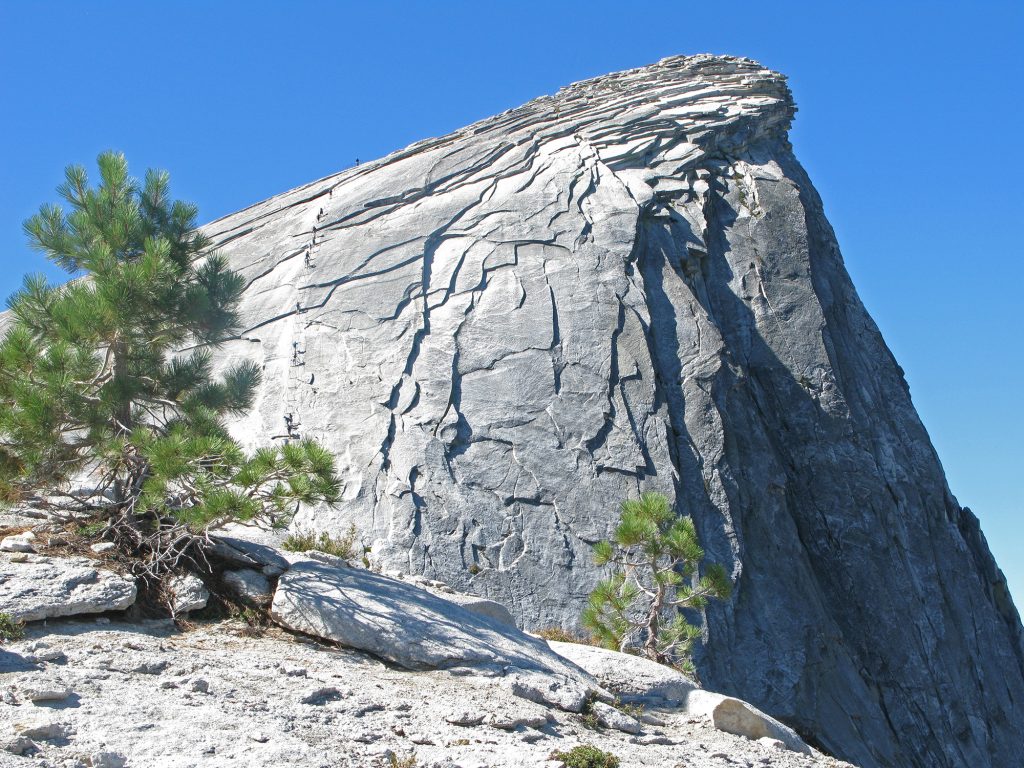
Pressure release is particularly evident when we look at intrusive igneous rocks. Most intrusive igneous rocks cooled down from very high temperatures deep under the Earth under significant pressure. As erosion goes on, and overlying material is removed, those rocks come up towards the surface where the pressure is less. The pressure release helps to cause those granites to crack into sheets parallel to the Earth’s surface, in a process called sheeting or [pb_glossary id=”3961″]exfoliation[/pb_glossary].
Biological effects
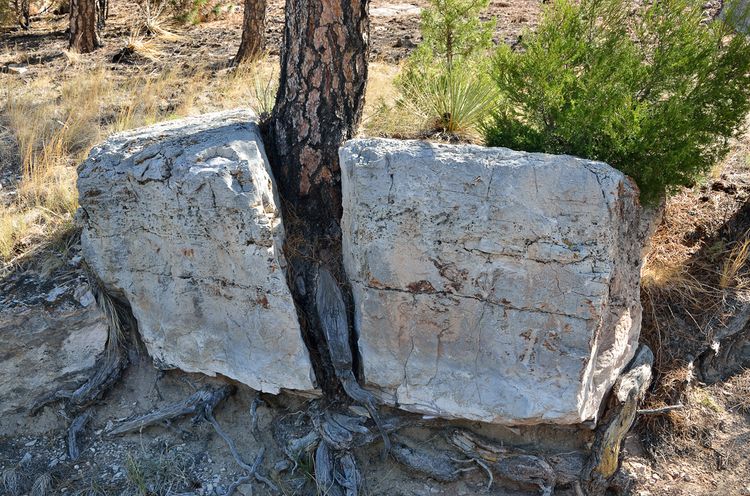
Biological processes also contribute to mechanical weathering. For example, plant roots can penetrate deep into cracks in rocks and help to wedge them apart. (Of course, plant roots also secrete chemicals to extract nutrients, thereby contributing to chemical weathering.)
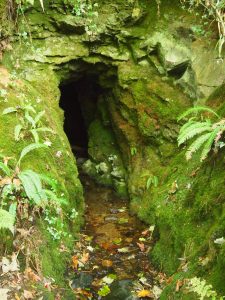
Animals that burrow into soft rocks are also mechanical weathering agents.
Perhaps the most effective of all are humans. Mining and quarrying, highway construction projects and excavations for building foundations and basements all lead to significant mechanical weathering of the Earth’s crust!
Daily temperature change as a cause of physical weathering?
Daily temperature change has often been proposed as a cause of mechanical weathering in desert environments, where there is a large temperature change between day and night. This hypothesis stems from observations following forest fires. Rocks that have undergone rapid temperature changes of hundreds of degrees in fires can show exfoliation.
The desert environment is one where we would expect temperature change to be most effective. We would also expect chemical weathering to be slow in the desert because of the lack of water. (W water is essential to most chemical weathering.)
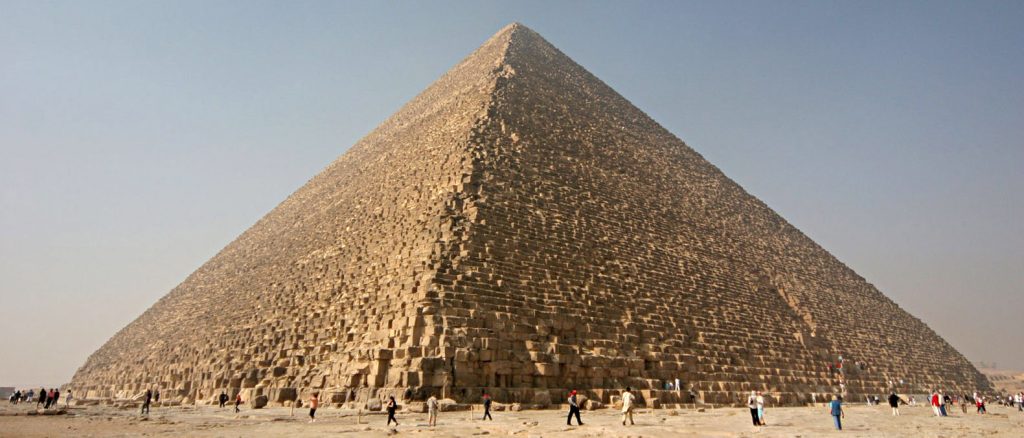
So, we might predict that the sunlit sides of desert ancient monuments would weather more quickly because the temperature change is greater. However, observations of these monuments show that it is the shaded surfaces that have weathered more quickly. It seems that even relatively slow chemical weathering, promoted by dew on these cooler surfaces, is faster than any damage resulting from heating on the sunlit side.
Our conclusion, then, is that temperature change (except in areas affected by forest fires or volcanic activity) is probably a much smaller part of physical weathering than was once thought.
Chemical weathering
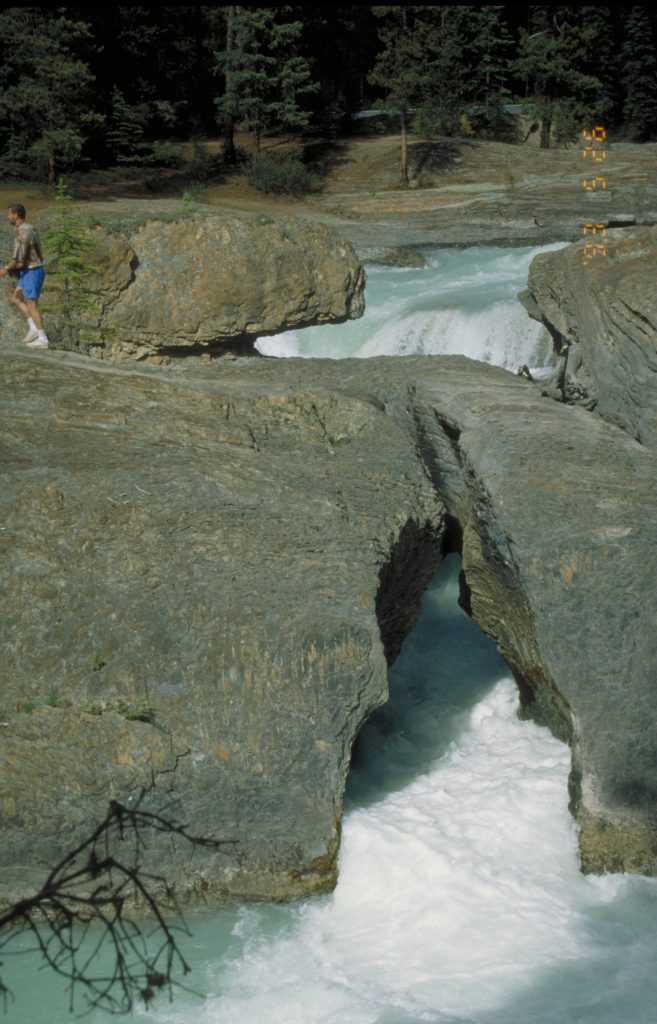
Chemical weathering involves chemical changes to the minerals and rocks exposed at the surface of the Geosphere. The raw materials for chemical weathering are
- Gases from the atmosphere, particularly carbon dioxide, although sulfur dioxide can play a role in chemical weathering as well.
- Water is almost always involved, and you can read more about the role of water in the chapter dedicated to the behaviour of water.
- Minerals and non-mineral solids in the rocks of the Geosphere
- New minerals
- Ions in solution
For example, potassium feldspar, a major component of granite, may be broken down in a hydrolysis reaction which can be written in words as:
Feldspar + hydrogen ions + water => kaolinite + potassium ions + dissolved silica
or in symbols as:
2KAlSi3O8 + 2H+ + H2O => Al2Si2O5(OH)4 + 2K+ + 4SiO2
Kaolinite is an example of a clay mineral. It is a sheet silicate, but like most clay minerals, kaolinite is typically very fine-grained — only a few microns (thousandths of a millimetre) in diameter. Clay minerals typically have to be identified by a technique called x-ray diffraction. Kaolinite is a typical solid product of silicate weathering.
There are other products of chemical weathering that end up dissolved in water. In the above reaction, these are the potassium ions and some dissolved silica. A huge range of ions are present in most river waters, resulting from chemical weathering in their catchment areas.
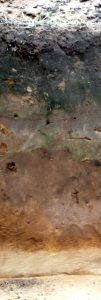
Regolith and soil
Solid material that remains at the site of weathering is known as regolith, or more commonly, as soil. What’s the difference? Soil is a mixture of the solid products of weathering and carbon-bearing compounds produced by the decay of plants. Most soils are products of extremely complex interactions between solid minerals, animals and plants, water, and dissolved materials.
So on Mars, where there is weathering but (so far as we know) no biosphere, the surface products of weathering cannot be described as soil; regolith is the appropriate term. On Earth, except in extremely cold and extremely dry environments, regolith is mostly soil.
Sediment and sedimentary rock
Clastic sediment and sedimentary rock
Clastic sediment is sediment composed of solid products of weathering. It’s important to note that, although “clastic” literally means “broken”, clastic sediment includes not just the broken fragments produced by physical weathering; it also includes solid products of chemical weathering, principally clay minerals.
Recently deposited clastic sediment tends to consist of loose particles, but over time the particles may become cemented together to produce more solid rocks. The processes of change that sediments undergo after deposition, are called diagenesis. We will look at diagenetic processes in more detail once we have concluded a tour of the most common types of sediment and sedimentary rock.
Transport of clastic sediment
Transport processes
Solid particles of sediment are moved over the surface of the Geosphere by a number of processes. We can recognize:
Most of these processes are described in other sections of this book: transport by gravity and by water are described in sections on mass transport and on erosion by liquid water; transport by ice is described with glaciers, and transport by wind is described in the section on the atmosphere.
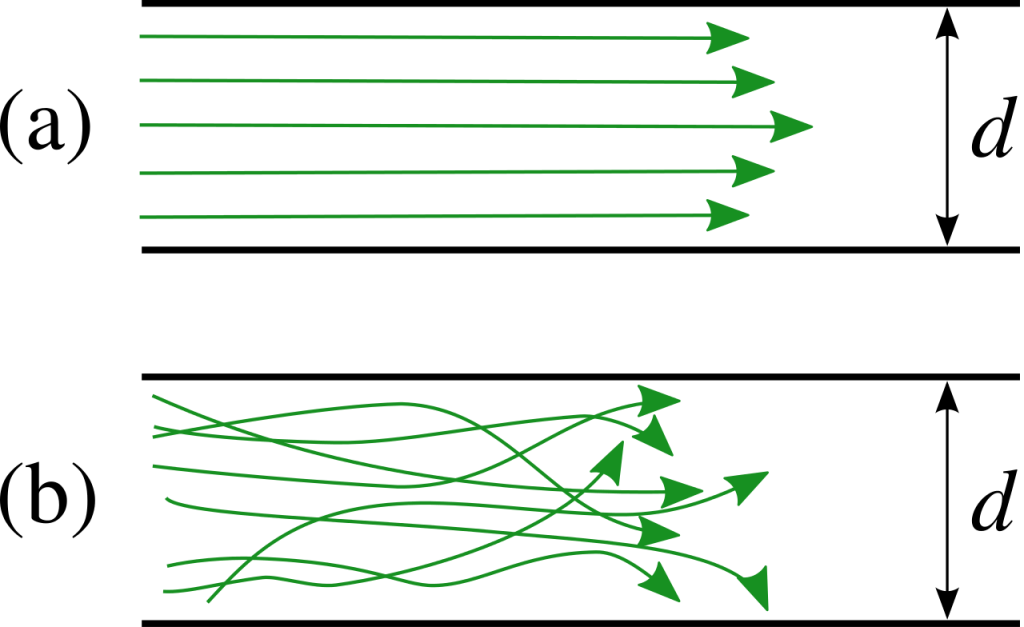
One important feature to note is that flow of water and air is normally turbulent whereas mass transport driven by gravity can be laminar, and the flow of ice is always laminar. Turbulent flow is much more effective at moving small particles than large particles, and for any given speed of flow, there is a maximum size that can be transported. Therefore, wind and water flow are effective at sorting sediment into specific sizes. Typically coarse particles are deposited where the current is fast, whereas deposits of very fine sediment indicate that the current was slow. In contrast, sediment deposited by ice or by gravity-driven mass flow is usually not sorted.
Grain-size and sorting are therefore important indicators of the environment of transport deposition for clastic sediment.
Grain-size
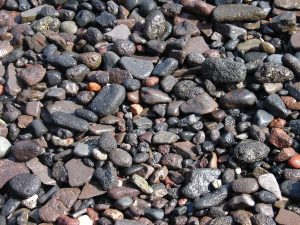
There are many classification schemes for the grain size of clastic sediment. In this book we use the simplest possible scheme, dividing sedimentary particles, or clasts, into three large categories.
- Gravel: Coarser than 2 mm. High-energy environments.
- Sand: Finer than 2 mm but coarser than 1/16 mm. Moderate-energy environments.
- Mud: Finer than 1/16 mm. Low-energy environments. Mud may be further subdivided into:
(Note that this use of the word “clay” is a little different from our use of the term in mineralogy to describe a class of minerals, mainly sheet silicates produced by chemical weathering. The word “clay” is used with both meanings, because in practical terms, most particles finer than 4 μm are in fact clay minerals.)
Sorting
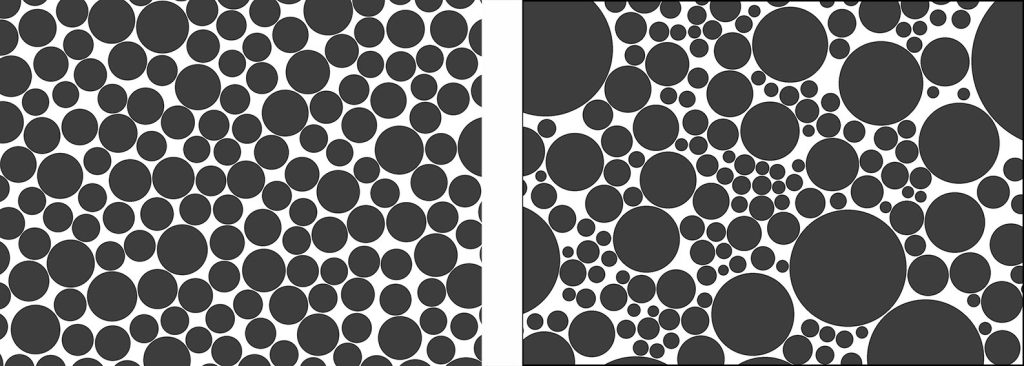
The sorting out of sediment particles by the flow of wind and water takes some time. As a result, we can use sorting as an indication of the length or repetitive nature of sediment transport. For example, on beaches, where wave action moves sediment particles repetitively over long periods of time, the sand grains in any one place tend to be almost exactly the same size: we say the sediment is well sorted. In contrast, sand that is newly eroded, found in the steep upper reaches of river systems, tends to have a range of grain sizes and is therefore poorly sorted.
It’s important to know that glacier flow does not sort sediment. Glacier flow is laminar, and sediment is suspended by the strength of the solid ice, not by the turbulent eddies that occur in water and air flow. Therefore, till deposited by glacial ice is some of the most poorly sorted sediment. (Traditionally in British glacial geology, till was known as boulder clay, reflecting the fact that the coarsest and finest sediments were mixed together.)
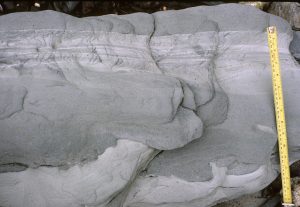
Gravity flow of sediment may or may not achieve sorting. In a debris flow or mud flow, larger particles are supported by the strength of a mud-rich slurry, somewhat similar to a glacier, so the resulting sediment is poorly sorted. On the other hand, the submarine avalanches known as turbidity currents that flow down underwater slopes are turbulent, so they do achieve a small amount of sorting. Coarse grains are deposited first and finer grains settle out later, forming a graded bed. Nonetheless, because turbidity currents are short-lived (typically lasting a few hours at most) they are not very effective at sorting sediment, so turbidity current deposits tend to be poorly sorted overall, .
Well sorted sands can have very high porosity, especially if the grains are rounded. The large spaces between the grains can hold water or hydrocarbons. Well sorted sands are therefore sought by humans as sources of water (aquifers) and as reservoirs of petroleum (oil and natural gas). In poorly sorted sands, the small grains tend to fill the gaps between the larger grains, so both porosity and permeability are low.
Clastic sedimentary rocks
Conglomerate
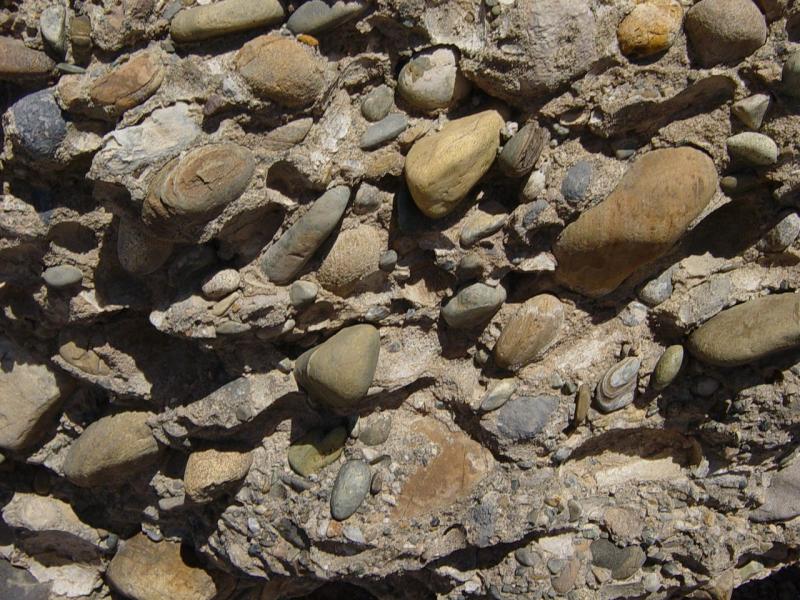
Sedimentary rock formed from gravel is known as conglomerate. Conglomerate is deposited in high-energy environments ranging from fast-flowing rivers to submarine slopes. Often, it’s possible to recognize the rock-types in the clasts of a conglomerate without using a microscope.
(Conglomerate may have angular or rounded clasts, or a mixture. Some textbooks recognize angular conglomerate with a separate name breccia. However, “breccia” has other meanings. We reserve the name “breccia” for rock that has been broken into angular pieces by the movement of faults, or the action of volcanic explosions.)
Sandstone
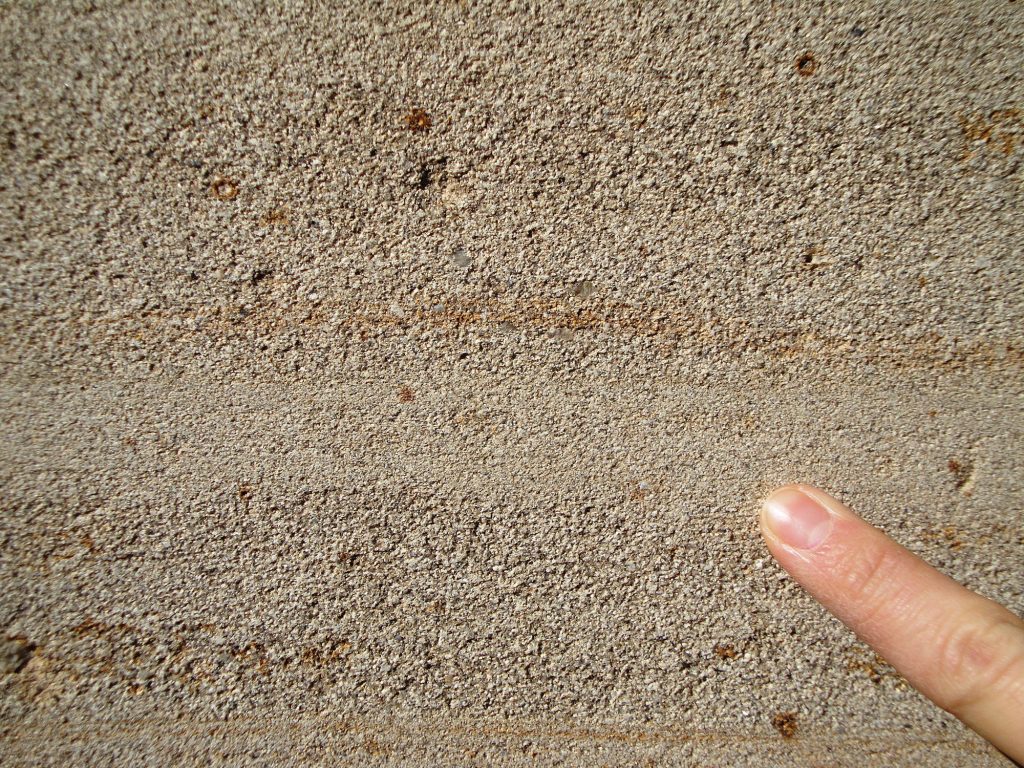
Sandstone is clastic sedimentary rock with an average grain size between 2 mm and 1/16 mm, representing moderate-energy environments. In contrast to igneous rocks, which have tightly interlocking grains, sandstone usually shows clastic texture, in which the grains rest against one another without interlocking. Well sorted sands typically represent environments of long or repeated transport by wind or waves. Well sorted sandstones can have very high porosity, especially if the grains are rounded. The large spaces between the grains can hold water or hydrocarbons. Well sorted sands are therefore sought by humans as sources of water (aquifers) and as reservoirs of petroleum (oil and gas). In poorly sorted sands, the small grains tend to fill the gaps between the larger grains, so porosity and permeability are low.
Mudstone
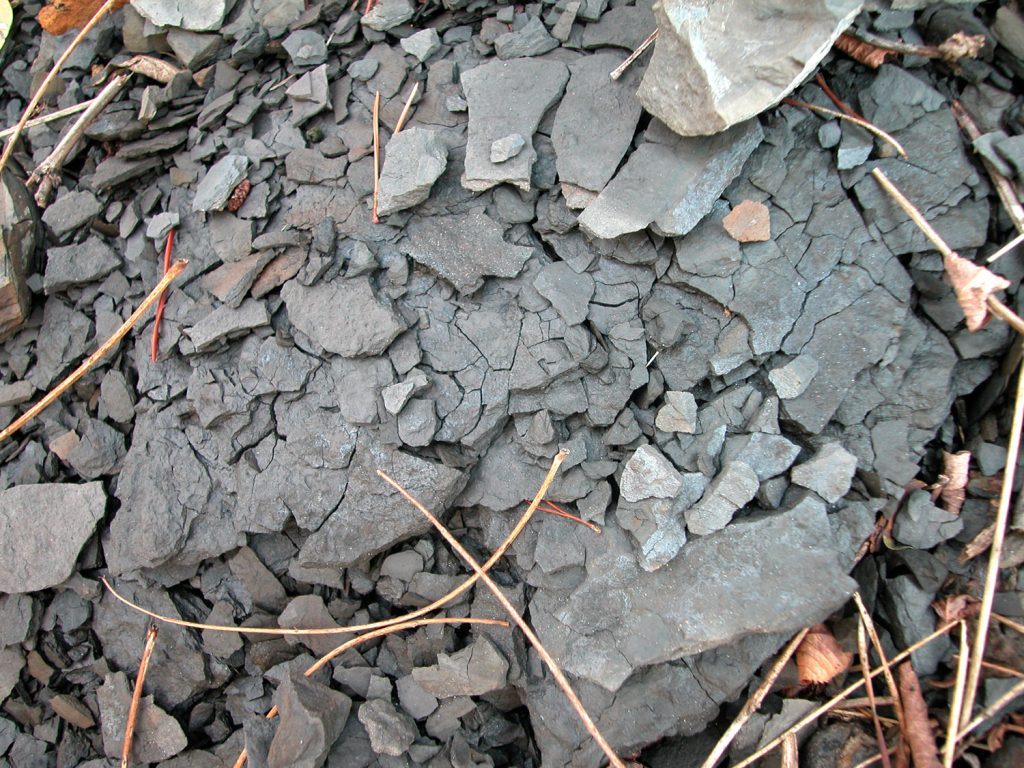
Mudstone is clastic sediment with grain size below 1/16 mm. Mudstones represent low-energy environments, including lake floors, floodplains, and sediments deposited at high or low tide on sheltered shorelines and in estuaries. Mud is widely deposited on the ocean floor, too.
Most mudstone is composed, at least in part, of very fine clay minerals. Because clay minerals are sheet silicates, they can very easily become aligned during compaction of mud in a sediment pile, producing a rock that displays fissility – it splits easily into thin flakes. Mudstone that has this property is called shale. Because fissility is so common in fine-grained sedimentary rocks, most mudstone can also be described as shale.
Colour of clastic rock
Clastic sedimentary rocks, especially mudstones, can display a wide range of colour. In general, sediment that contains a lot of residual organic matter appears black. Organic-rich mudstone passes gradationally into impure coal. In contrast, mudstone without organic matter commonly appears red, because of the presence of fine grained hematite, an oxide of iron. Red colour is also common in sandstone and conglomerate. Such rocks, known as “redbeds” may be indicators of deposition in oxidizing conditions on land, particularly in arid environments where not much organic matter is buried with the sediment. However, this correlation between colour and environment of deposition is not universal. Other evidence must be found before concluding that a particular sediment was deposited on land or under the sea.
Non-clastic sedimentary rock
Non-clastic sedimentary rocks are those derived from the dissolved products of weathering, together with other products of the biosphere. One category — the carbonate sediments — is really abundant. The others are much less common but almost all have been important materials for humans at one time or another.
Carbonate sediment and limestone
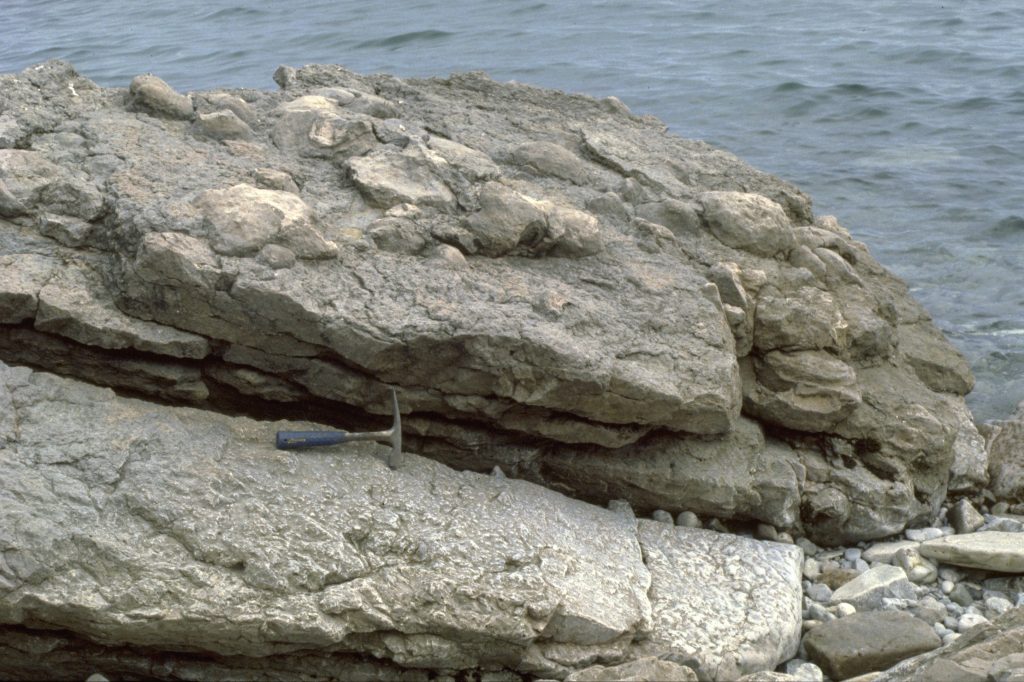
Carbonate sediments, as the name implies, contain carbonate ions, and by far the most important carbonate mineral is calcite. A polymorph, aragonite is produced by certain organisms, but it tends to convert to the more stable form calcite once it’s buried in sediment. Both minerals are calcium carbonate, with the formula CaCO3. A rock made of calcium carbonate is known as limestone.
Both calcium and carbonate ions are present in sea-water, and although it is theoretically possible to produce a carbonate deposit by evaporating sea-water, the vast majority of carbonate sediments are produced in some way by the action of the Biosphere. (An exception is dripstone formed in caves by direct evaporation of groundwater.)
In some cases limestones are obviously built of fragments of fossil organisms, but in other cases limestones consist of fine grained calcium carbonate called lime mud.
Regardless of the texture, limestone typically indicates a marine environment of high organic productivity, and carbonate sediments are most abundant in warm, tropical continental shelf seas. In some such areas, carbonate sedimentation is able to keep pace with subsidence of the continental margin, producing a carbonate platform where shallow water is underlain by carbonate sedimentary rock many kilometres thick.
Carbonate sediments are also produced by planktonic animals and plants in the open ocean, so slowly deposited sediment (known as calcareous ooze) is common on the deep ocean floor. Chalk is a soft limestone formed from ancient calcareous ooze primarily composed of tiny oval plates called coccoliths, formed unicellular phytoplankton (coccolithophores).
Limestone is used by humans as a building material both as raw rock and in the manufacture of cement, which is made by roasting limestone together with silicate sedimentary rock like mudstone.
Organic sediment and coal
Not to be confused with carbonate sediments, in which the carbon is oxidized, are carbonaceous or organic sediments, composed of the reduced carbon remains of living organisms. After diagenesis, sedimentary organic matter may be converted to hydrocarbons — coal, oil or natural gas. The burning of hydrocarbons replaced wood-burning as humanity’s principal source of energy during the industrial revolution in the 1700s CE, and human combustion of hydrocarbons has increased steadily ever since.
Peat
The largest accumulations of organic matter occur in peat, the accumulated remains of land plants, typically found in wetlands, or mires, in low-lying areas of the continents, and in marginal-marine environments like deltas. Once dried, peat may be burned, and it is used as a fuel in some parts of the world.
Coal
As peat is buried beneath accumulating sediment, it undergoes compaction to a fraction (typically around one eighth) of its original volume, and loses components such as water, hydrogen, oxygen, and some of the carbon. The volatile materials may escape and accumulate within porous rocks elsewhere in the Geosphere in natural gas. The remaining solid black rock is coal.
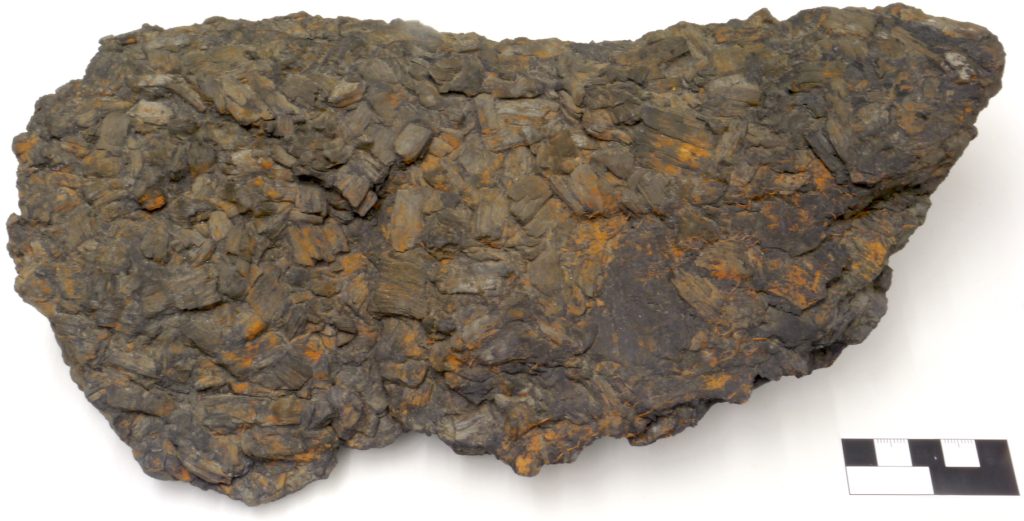
Different ranks of coal are recognized, based on increasing content of carbon (and increasing burning temperature).
- Lignite 25-35% carbon
- Subbituminous coal 35–45% carbon
- Bituminous coal 45–86% carbon
- Anthracite 86–97% carbon
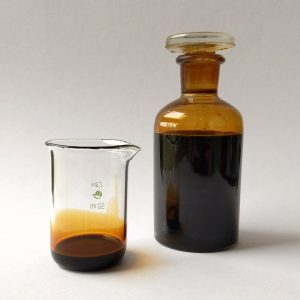
Oil
In contrast to woody land plants, which tend to produce solid (coal) and gaseous (natural gas) hydrocarbons, the accumulated remains of microbial organic matter may produce liquid hydrocarbons — oil — after diagenesis. Oil may remain in the fine-grained sedimentary rock in which it formed, forming oil shale, or it may leak out into more porous, permeable rocks like sandstone or limestone, forming an oil reservoir.
Siliceous sediment
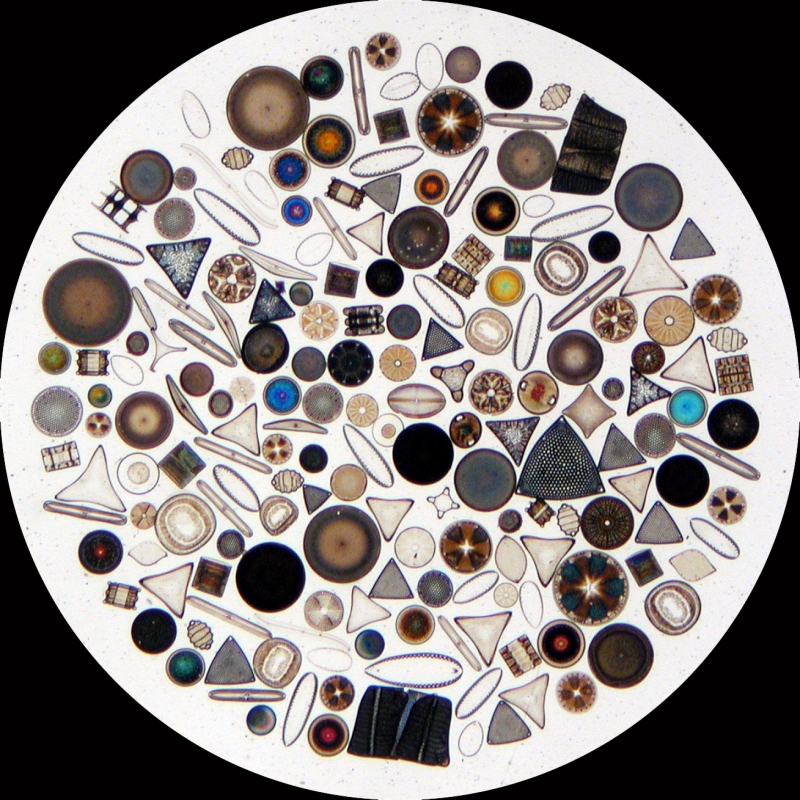
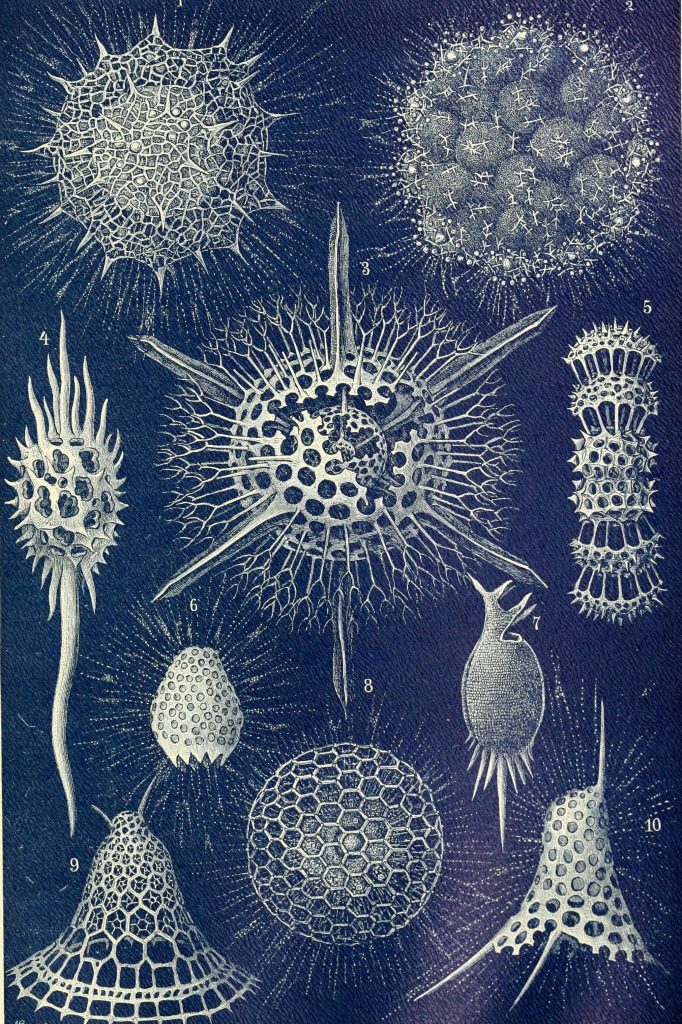
Some unicellular phytoplankton (diatoms) and zooplankton (Radiolaria) that live in the surface waters of the oceans and (in the case of diatoms) some lakes have evolved the ability to build skeletons from silica (SiO2) extracted from sea-water. The skeletons are not quartz; instead they are a glass-like disordered, non-crystalline form of silica called opal, which settles on the deep ocean floor to produce siliceous ooze. Dried-out, this material is sold as diatomaceous earth for pest control in plant care. Opal is also formed in small amounts by some sponges that live on the sea-floor. Opal can also form from hot (hydrothermal) water flowing through newly formed igneous rock.
During diagenesis, the silica in opal may be redistributed and converted to a hard rock called chert, made of fine-grained quartz; because of the tightly interlocking grains chert typically has hardness close to 7 on Moh’s scale, the hardness of pure quartz. Like quartz, chert may show conchoidal fracture, and breaks to form very sharp edges. For this reason it was very important for early human technology, being the principal tool-making material during the stone age before metals were developed by humans.
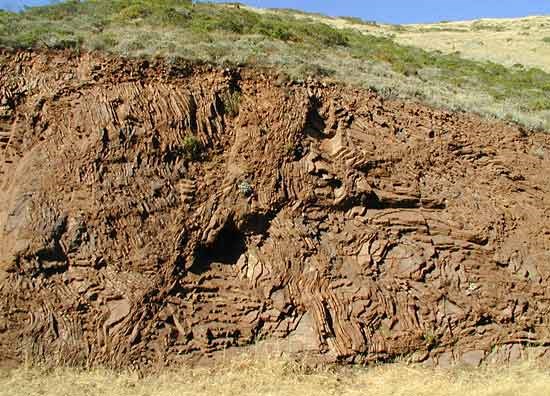
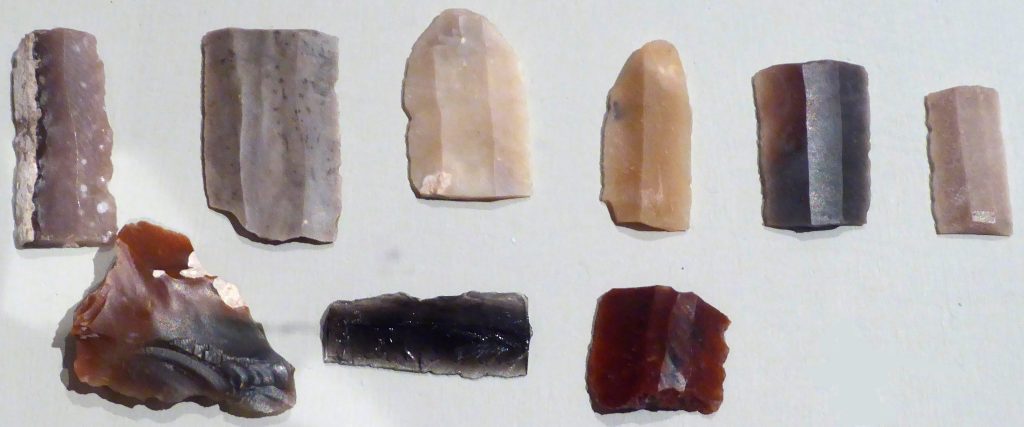
Iron-rich sediment
Iron-rich sediments occur in the sedimentary record of the Earth’s crust in many places and at many times, and they are humanity’s principal source of iron (the main component of steel). but there appears not to be a present-day environment in which iron-rich sediments are deposited in quantity. Thus it’s more difficult to use actualism — the present as a key to the past — to interpret iron-rich sediment than it is other types of sedimentary rock.
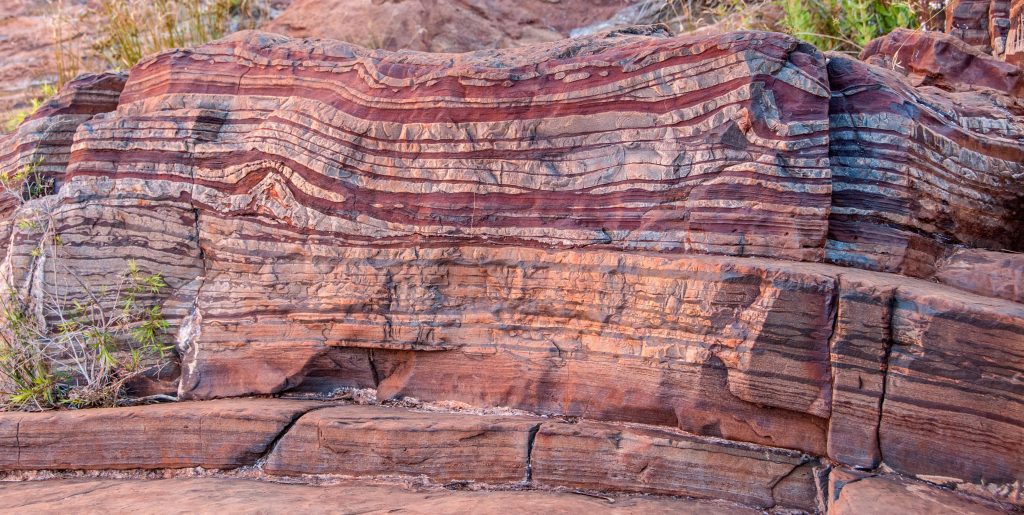
The largest category of iron-rich sediments are banded iron formations formed in Precambrian time, during the Archean and Proterozoic eons. They contain a variety of iron-rich minerals: oxides, carbonates, sulfides, and silicates, that are typically interbedded with chert to form spectacularly layered rock.
Although we do not have a modern analogue for banded iron formations, it appears that many were formed during the early Proterozoic great oxygenation event, when oxygen first began to appear in the Atmosphere. Reduced iron (II) or Fe2+ ions are very soluble in water, whereas the removal of an electron (by oxygen) to make iron (III) or Fe3+ ions makes iron much less soluble. It is hypothesized that the Archean oceans contained much more dissolved iron than at present day, and that the early Proterozoic rise in atmospheric oxygen led to the deposition of large amounts of iron in sediments.
Evaporite deposits
The dissolved load of rivers ends up in the sea, and when water returns to the land during the operation of the hydrologic cycle, those dissolved ions remain in the sea, tending to increase its salinity — the concentration of dissolved ions — over time. However, some ions are removed from the sea by biological or other processes. Calcium ions, for example, are constantly delivered to carbonate sediments by living organisms, while dissolved silica (SiO2) is removed by diatoms and Radiolaria. The result is that the most abundant ions left over in sea-water are sodium (Na+) and chloride (Cl–) ions, which make sea-water taste of salt. The next most abundant ions are Magnesium (Mg2+), Sulfate (SO42-), Calcium (Ca2+) and Potassium (K+).
When sea-water is intensely evaporated, these ions come out of solution. Surprisingly, halite (sodium chloride) is not the first to form, because although sodium and chloride ions are most abundant, sodium chloride is extremely soluble. Typically, the first evaporite mineral to come out of solution is gypsum, hydrated calcium sulfate CaSO4.2H2O, which starts to appear when about two thirds of the water has been evaporated from normal sea-water. Halite, sodium chloride NaCl, appears when 90% of the the original water has been evaportated. After that, a number of potassium-bearing evaporite minerals may appear as the remaining sea-water, now termed brine, is evaporated.
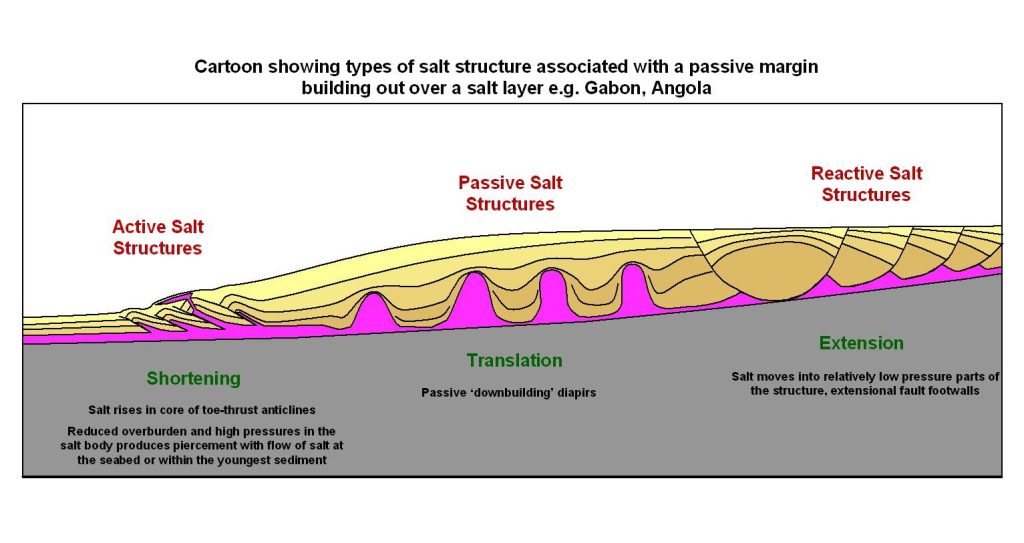
![]() |
![]() |
The resulting evaporite rocks are rock salt (a rock made mostly of halite), gypsum (the same word is used for the mineral and the rock), and potash. All these rocks have very low density, and are very soft. They flow within the Earth’s crust when subjected to the load of overlying, denser sediment, forming a variety of complex structures, including salt domes — vast pillars of evaporite rock sometimes kilometres high within the Earth — and sometimes even salt glaciers — flows of salt that emerge at the land surface in very arid environments.
Sediment to rock: Diagenesis
Sedimentary rocks are typically composed of minerals and other substances that are relatively stable at the Earth’s surface, but once sediments are buried, conditions change, and the sediment starts to be modified. These changes are called diagenesis. Diagenetic changes that convert loose sediment to solid sedimentary rock are known as lithification.
The boundary between diagenesis and metamorphism (covered in another section) is gradational and rather poorly defined, but temperature conditions below about 175°C, and pressures below 300 MPa are generally described as diagenetic. Higher pressures and temperatures are generally characterized as metamorphic.
Diagenesis involves a number of different processes, both physical and chemical.
Compaction
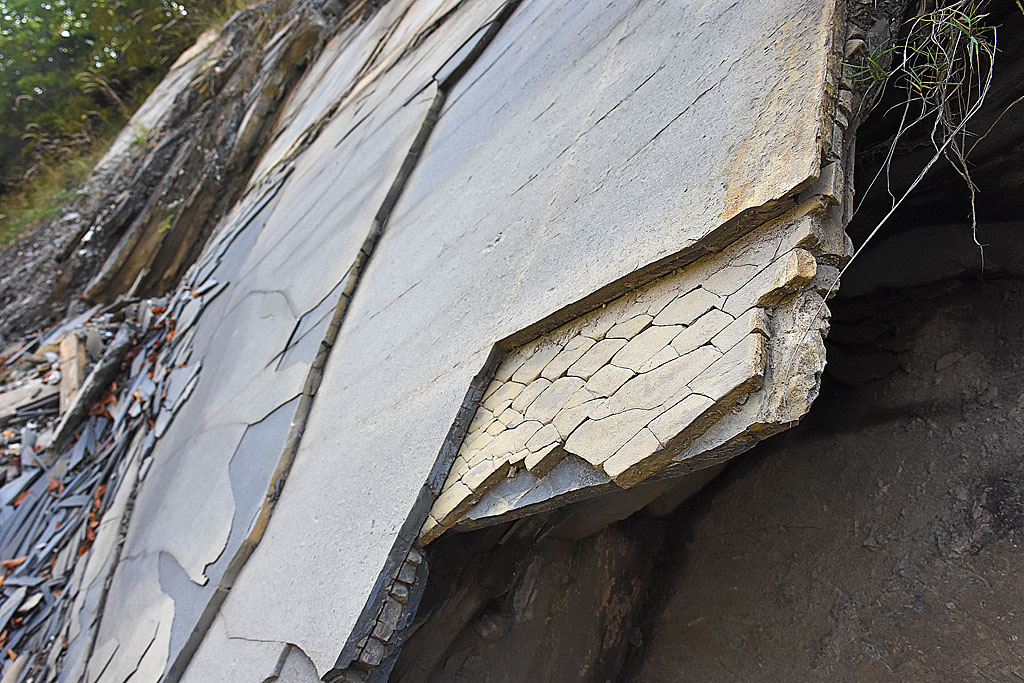
During compaction, water is driven out by weight of overlying sediment. Compaction has a particularly conspicuous effect on muds, because most of the mineral grains are sheet-silicate clay minerals that trap large amounts of water as mud is deposited. As the water is squeezed out during compaction, the clay mineral flakes all become aligned horizontally, giving the compacted rock a sedimentary fabric called fissility. Mudstone that shows fissility is called shale.
Solution
Mineral grains may dissolve during diagenesis in response to chemical conditions or pressure, which affects the solubility of many minerals. Solution tends to be particularly effective where grains are pressed against one another. It may cause grains that were formerly loosely touching to become knitted tightly together by pressure solution.
Cementation
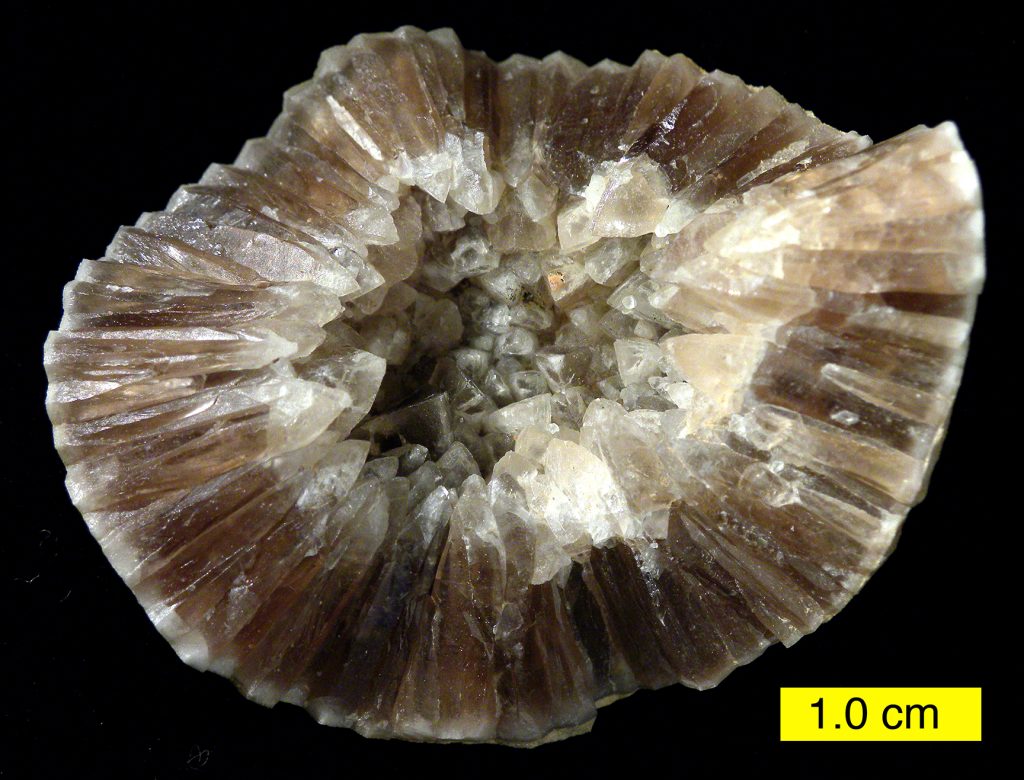
Cement is any mineral deposited in pore space. The most common cement minerals are calcite and quartz, but almost any mineral stable in near-surface conditions can occur as cement. Note that the term cement includes any mineral deposited in pore space, regardless of whether it actually causes lithification. For example, in a limestone, the calcite that forms inside a fossil shell may play no part in glueing that shell into the rock, but it’s called cement just as much as calcite that occurs between the fossils.
Chemical reactions
Many different chemical reactions can occur during diagenesis, converting one mineral to another, or changing the composition of (for example) solid organic matter to produce liquid or gaseous hydrocarbons. Some common examples are
- Conversion of unstable polymorphs and non-mineral materials to stable minerals:
- Aragonite is converted to calcite
- Opal is converted to quartz
- Volcanic glass is converted to clay minerals
- Maturation of hydrocarbons: peat is converted to coal and organic matter derived from microbes (kerogen) is converted to oil and natural gas.
The breakdown of rock material at the Geosphere surface due to the action of the Atmospher, Biosphere, and or Hydrosphere.
The removal of material from a site of weathering on the Earth's surface.
The envelope of gas surrounding a planet
All the free water on Earth, in the forms of water vapour, liquid water, and solid ice.
Weathering that forms fragments of rock, without chemical change.
Changes in the mineral composition of rocks as they are exposed to air and water at the Earth's surface, producing new minerals and dissolved material
Weathered material that remains in its original position, without removal by erosion. Regolith mixed with plant material is called soil.
A mixture of weathered, fragmented rock and organic matter at the surface of the Geosphere
Material derived ultimately from weathering, that is transported and/or deposited as grains on the surface of the Geosphere
The mechanical weathering effect of water in thin cracks as it freezes to form ice, splitting rocks apart as a result of expansion.
Physical weathering caused by contact between moving rock fragments and larger rock surfaces
The cracking of previously buried rocks into sheets as they are brought close to Earth’s surface
The splitting of solid rocks into sheets roughly parallel to the surface, due to volume changes and stresses during uplift, weathering and erosion
An abundant group of framework silicates containing aluminum together with some combination of
potassium, sodium and calcium
A reaction involving the breakdown of a mineral through the action of water which provides hydrogen and hydroxyl ions.
Sediment formed from the solid products of weathering
Clay mineral: A fine-grained hydrous mineral; clay minerals are mainly sheet silicates, most characteristically
formed by chemical weathering
A change in sediment after it is deposited
Having turbulence: wirling movements in a fluid.
Sheet-like; applied to flow of fluids, it describes flow along sub-parallel lines, lacking the gyres typical of turblence.
The average diameter of a particle within a rock.
The process in sedimentation wherein particles are separated on the basis of size, or the degree to which the particles in a sediment have been sorted, producing a narrow range of sizes.
Sediment consisting of particles larger than 2 mm in diameter.
Clastic sediment with a grain-size finer than 2 mm but coarser than 1/16 mm
Sediment composed of particles with a diameter less than 1/16 mm.
Mud particles with diameters between 1/16 mm and 1/256 mm (or 4 μm)
Sedimentary deposits with particles of about the same size.
Having a very wide range of grain sizes
The downward movement of a sediment-water mixture due to gravity. Debris flows and deep-sea turbidity currents are examples of gravity flows.
A turbulent flow of sediment-rich water moving down slope under clearer water due to the action of gravity.
A bed which has coarse sediment at the base but becomes progressively finer-grained towards to top..
The proportion or percentage of space in a solid that may be filled by fluids.
Unit within the Earth's crust that is a reservoir of groundwater usable by humans
Naturally occurring liquid and gaseous hydrocarbons: Oil and natural gas.
The ability of a solid to and allow fluid to move through it.
Coarse clastic sedimentary rock composed of gravel-size (>2 mm) grains.
Rock with gravel-sized angular grains
Clastic sedimentary rock formed from lithified sand (sediment with grains finer than 2 mm, but coarser than 1/16 mm)
The texture of clastic sedimentary rocks, where individual grains rest against each other without
interlocking
Clastic sedimentary rock with a grain-size below 1/16 mm
Reduction in volume of a sediment or soil due to the expulsion of air or water
The ability of a rock to split into thin flakes or sheets parallel to sedimentary layers.
Mudstone that is fissile (ie with clay minerals aligned due to compaction causing it to break into thin flakes.
Matter containing reduced carbon that originated in a living organism.
; A mineral form of oxidised iron (Fe₂O₃)
Negatively charged ions with the formula CO₃ ²⁻; or, a substance containing such ions
CaCO3; the most common mineral form of calcium carbonate
A form of calcium carbonate CaCO3; that is produced by many organisms but is less stable than the more common polymorph calcite.
Sedimentary rock composed mainly of calcium carbonate
A deposit of calcium carbonate formed by evaporation of water in caves
Water that occupies spaces within the Geosphere
Remains of ancient organisms, buried in rocks
Fine-grained calcium carbonate
A flat continental shelf where the rate of carbonate sedimentation is able to keep up with
subsidence producing great thicknesses of shallow-marine carbonate rock
Deep-sea sediment comprising mainly the calcium-carbonate skeletons of foraminifera and coccolithophores
Soft limestone formed mainly from the calcite plates of unicellular planktonic plants called
coccolithophores.
Small oval scale of calcium carbonate secreted by a coccolithophore
free-floating plants
Unicellular phytoplankton that secretes plates (coccoliths) of calcium carbonate to cover their bodies. Chalk is formed from these plates.
A substance with a high content of reduced carbon
Any reduced carbon compound containing carbon–hydrogen bonds (or, rarely carbon–halogen bonds); elemental forms of carbon (with only carbon–carbon bonds) are regarded as inorganic.
A compound constructed from carbon-carbon and carbon-hydrogen bonds.
Carbon-rich sedimentary rock formed from solidified organic material that originated as peat
Liquid Petroleum; organic matter that has undergone burial and subsequently been transformed into hydrocarbon-rich liquid.
Hydrocarbon-rich gas derived from sedimentary organic matter.
Carbon-rich material formed from compressed plant remains; a first stage in coal formation.
Divisions recognized between coal types, based on increasing carbon content.
Fine-grained sedimentary rock which holds a large proportion of hydrocarbons.
A region within the Geosphere where liquid petroleum is present within porous, permeable rock.
Photosynthetic, unicellular plankton that inhabit oceans and lakes. Diatoms are notable for their siliceous skeletons of opal.
A group of unicellular marine plankton that construct silica skeletons that settle to the ocean floor
Non crystalline silica, sometimes including a small proportion of water; SiO₂·nH₂O.
Deep-sea deposits of fine grained sedimentary opal derived from organisms with silica skeletons such as diatoms and Radiolaria.
Primitive multicellular animals that lack true tissues and organs; most are filter-feeders
Hydrothermal describes groundwater that has been heated by geothermal energy
A hard rock composed of very fine interlocking grains of silica SiO2.
Silica SiO₂ in its most common mineral form.
A curved or ribbed shape, seen in the fracture surfaces of many minerals and a few rocks such as chert and volcanic glass
The principle that events in the past can be explained in terms of physical, chemical, or biological processes and laws that operate at the present day
Layers of iron-rich minerals and chert that were particularly common in the Proterozoic Eon
An eon early in Earth history prior to the Proterozoic from ~4 Ga to 2.5 Ga
The eon before the Phanerozoic, from 2500 to about 539 Ma, during which atmospheric oxygenation, the evolution of multicellular organisms, and Snowball Earth conditions occurred.
The mass emission of free molecular oxygen into the atmosphere by cyanobacteria at approximately 2.4–2.0 Ga.
Minerals or rocks produced by
precipitation of minerals from high-salinity water (such as seawater) during evaporation
A mineral form of hydrated calcium sulphate (CaSO₄·2H₂O)
The mineral form of table salt (NaCl)
Water having a salinity greater than normal sea water (35 parts per thousand)
The rock composed mainly of the mineral halite NaCl
Upwarped regions in the crust produced by rising columns of rock salt and other evaporite rocks
A flow of salt that emerges onto the Earth’s surface and then flows slowly down slope
The solidification of sediment into solid sedimentary rock
The transformation of a rock in the solid state due to high temperature and/or pressure.
Describes rock formed through transformation of older rock by heat and pressure, without melting
The structure of a rock defined by the preferred orientation of mineral grains or other components parallel to a line or plane, or both
The dissolution of mineral grains due to compressive stress.
Mineral matter deposited by groundwater in the pore space of a rock.
Minerals with the same chemical composition, yet a different structure.
Igneous rock formed by rapid cooling of lava, such that crystal lattices are unable to form; rock with the structure of a supercooled liquid.
The transformation over time of raw organic matter into fossil fuel.
Organic matter in sediment or sedimentary rock that is insoluble in organic solvents and that may form oil and gas if heated.