8 Rivers, Lakes, and Landscapes
This chapter is a pre-review version
In this chapter, we look at water on the surface of the continents, including rivers, lakes, wetlands etc. As we’ve seen from the section on the water cycle, about 40,000 cubic kilometers of water runs over the surfaces of the continents per year, and the total amount of surface water is around 190,000 cubic kilometers at any one time time.
Rivers have huge impacts on the landscape over time. They erode the channels and valleys in which they flow; they transport sediments supplied by weathering and erosion; and they deposit sediment in a variety of depositional landforms. Rivers are vitally important to humans as sources of water for drinking, agriculture, and industry, as sources of food, and as means of transportation.
Learning outcomes:
By the end of this section you should be able to:
- Define overland flow, base flow, stream flow, and discharge;
- Describe major features of drainage basins and river systems;
- dentify processes and features of erosion and deposition in river systems;
- Evaluate flooding risks associated with rivers;
- Interpret the formation of open and closed lakes.
Overland and stream flow
Onland water starts its journey as rain or snow falling on the land surface. Snow may be locked up in glaciers for periods lasting from hours to millions of years, but eventually it joins rainwater on the surface of the land. If we look at new rainfall or meltwater, we can distinguish water that travels as unconfined sheets — overland flow — from water that travels in confined channels — stream flow.
Overland flow
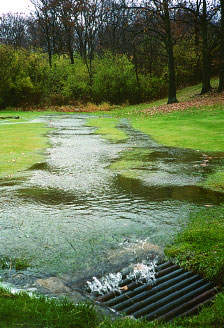
Overland flow doesn’t start immediately when rain falls or ice melts on the landscape. The first water in most cases soaks in to any porous material — regolith, soil, or porous rock — that is present at the surface of the Geosphere. The soaked-in water becomes groundwater, the subject of a later section. Once the ground is saturated, if the supply of rain or meltwater is sufficient, sheets of water start to accumulate on the land surface and move down-slope towards lower ground. This is overland flow. In most areas, it requires very heavy rain for overland flow to be visible, but in built-up areas where the ground is covered with concrete or asphalt, and in areas of naturally impermeable rock, overland flow may occur during moderately heavy rain.
Stream flow
In most environments, however, overland flow is fastest on locally steep slopes, and that leads to erosion, further concentrating the flow into gullies — small valleys — marking the transition to channellized or stream flow.
Water supply to streams
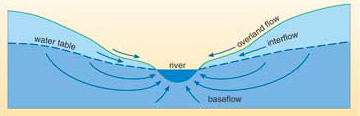
When we look at a stream it may not immediately be clear where the water is coming from. Certainly, overland flow is important in feeding many streams, but additional water — groundwater — enters most streams through the channel sides and channel flow. This component of water, that initially soaks in to the ground and enters a stream through its base, is known as base flow.
Therefore the total flow of water through a stream is approximately the sum of the amount entering as overland flow and the amount entering as base flow[1].
The rate of flow in a channel is determined by a number of factors, including the slope or gradient of the channel, the depth of water, and the roughness of the channel floor and sides.
Channel gradients
Channel gradients are extremely variable. Although they could be measured in degrees, many channels have extremely gentle slopes (fractions of a degree) so that gradients are more often measured as “metres per kilometre” or (particularly in the USA) in “feet per mile”.
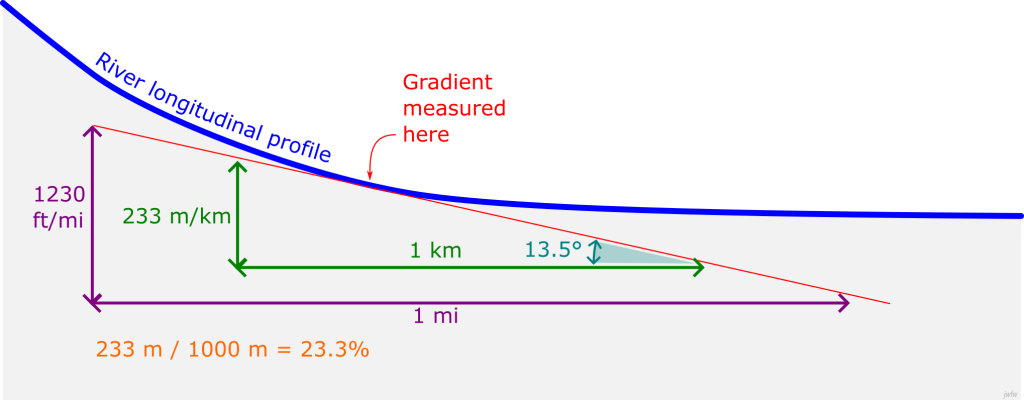
For example, the North Saskatchewan River in Edmonton, AB, Canada, where this text is being written, has a gradent of about 0.3 m/km which translates to about 0.17°. However, gradients are extremely variable. The steepest mountain streams may have gradients as high as 45°, or 1000 m/km. In contrast, downstream sections of the Mississippi have gradients around 0.01 m/km or 0.0006°.
In most river systems, the gradient is steep near the source, and becomes gentler downstream.
Flow velocity
The velocity of flow in streams is also very variable, both from stream to stream and even within a single channel. In a straight channel, the rate of flow tends to be fastest slightly below the water surface in the middle of the channel. The rate of flow is less near the bed of the channel because of drag against the channel floor, due to the viscosity of water and the roughness of the bed. The force per unit area, or stress exerted on the bed by the water, which moves sediment as bed load, depends on the velocity gradient (i.e. how much the speed of the water changes with depth). As a result, shallow, fast-moving streams can move large cobbles and boulders, whereas deep or slow moving streams are less likely to move large fragments of rock.
If a channel is sinuous (i.e. it has many bends) the momentum of the water causes it to flow fastest on the outside curve of each bend, and more slowly on the inside curve. Erosion tends to occur where the flow is fastest, on the outer bank, while deposition mainly takes place from slow-moving water on the inside of a bend.
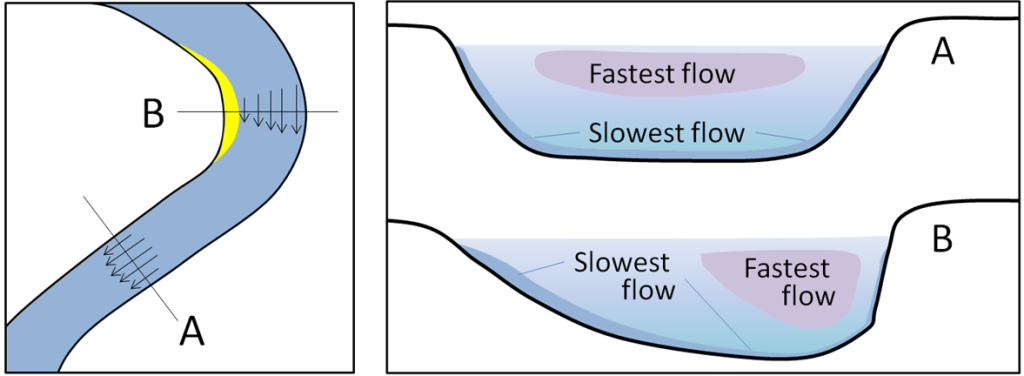
Discharge
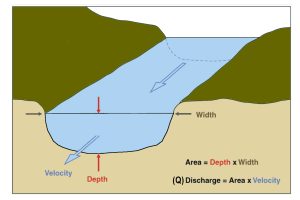
The amount of water that flows through a stream is called its discharge. Discharge is the volume of water that passes a given point on the stream in unit time: it’s usually given in cubic metres per second or m3/s or sometimes cubic kilometres per year km3/yr. If the average velocity of flow is known, then
discharge = velocity × cross-sectional area of stream
Q = vA
or
discharge = velocity × width × average depth
The discharge of the North Saskatchewan River in Edmonton, Alberta, is about 7 km3/yr or 220 m3/s[2].
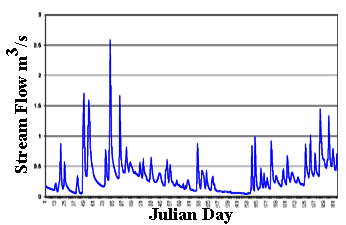
However, discharge tends to vary dramatically over time, especially with changing seasons. Hydrographs are a graphs of river discharge at one point in a stream, plotted against time, typically over periods of one to many years.
Hydrographs often show that short-term events such as large storms or snowmelt in the spring can lead to high discharge over short periods of time. These short pulses of high discharge may account for most of the sediment transport.
Drainage basins and river systems
To further explore the behaviour of streams, we need to look at how they are organized in river systems and drainage basins.
Small streams (tributaries) typically merge downstream into larger rivers. Smaller streams that flow into a larger rivers are known as tributaries of the larger river. The area drained by a major river and its tributaries is a drainage basin. Drainage basins are separated by drainage divides[3].
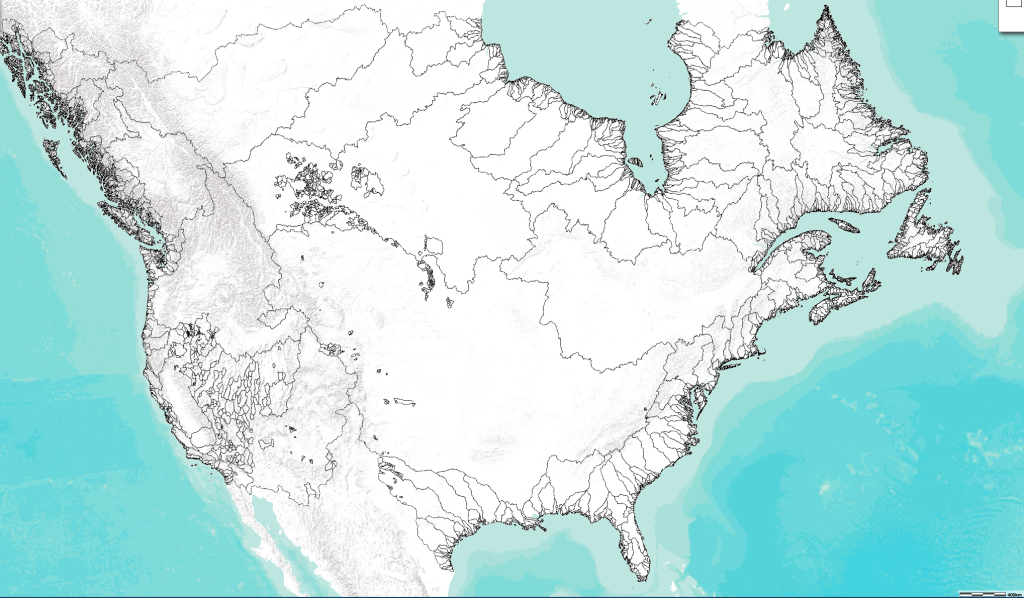
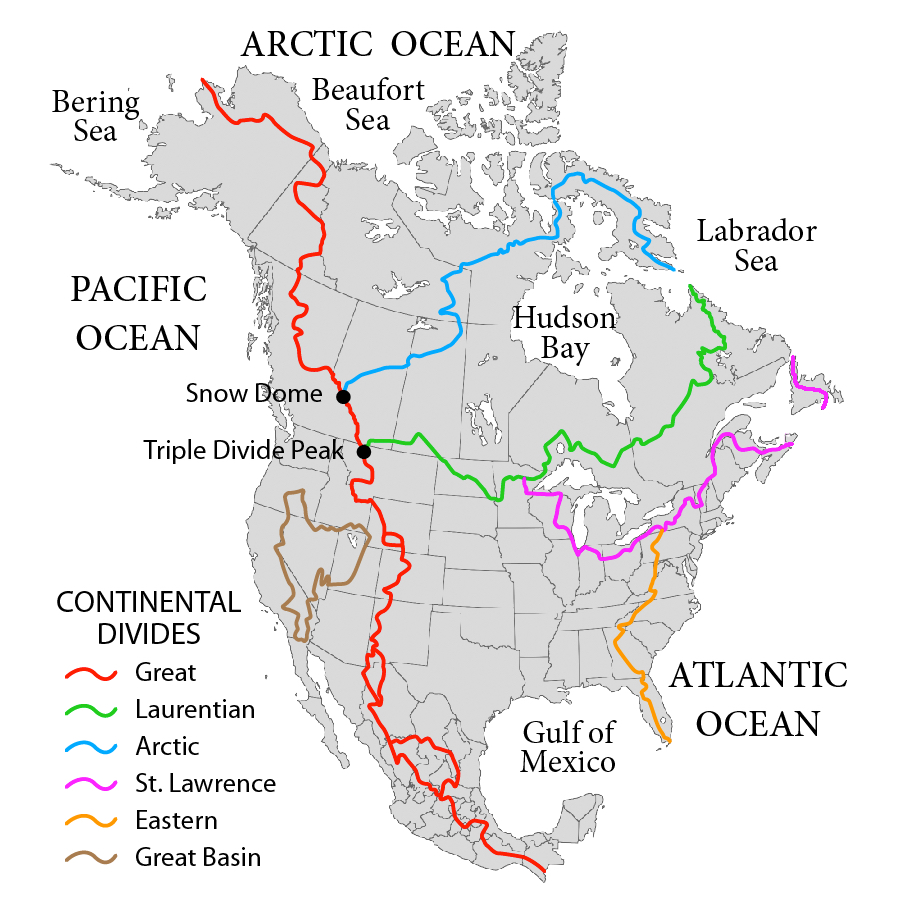
As tributaries join downstream, their discharge merges, so the discharge of individual channels rises, while the number of channels decreases. There are some exceptions where humans have modified rivers. In the map below, the discharge of the main channel of the Colorado River decreases downstream, because of very large human withdrawals of water for irrigation. Most of the withdrawn water re-enters the atmosphere through evapo-transpiration rather than entering the Gulf of California as it would have done in the natural state.
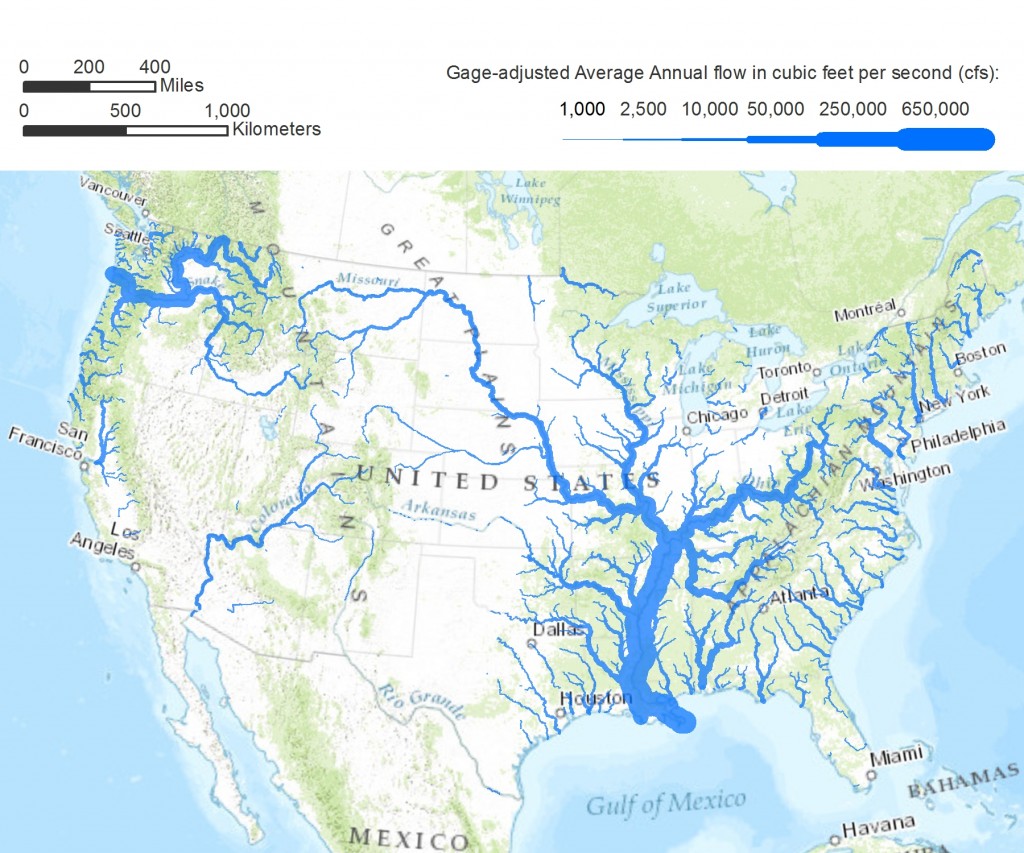
Much of the extra discharge in the downstream direction is accommodated by increases in both the width and depth of the channels, increasing the cross-sectional area. The average velocity of flow also tends to increase downstream, but more gradually. Despite this increase in velocity, the velocity gradient decreases because the width and depth are much greater. Downstream portions of large rivers are much less able to move coarse sediment particles.
Other features change from upstream to downstream. The slope tends to decrease downstream. If we plot a longitudinal profile of a mature river, showing elevation vs. distance downstream, we may see a curve from steep gradients upstream to very low gradients as the river approaches its base level, the elevation at which the river enters the sea, and the velocity of the river falls to near zero.
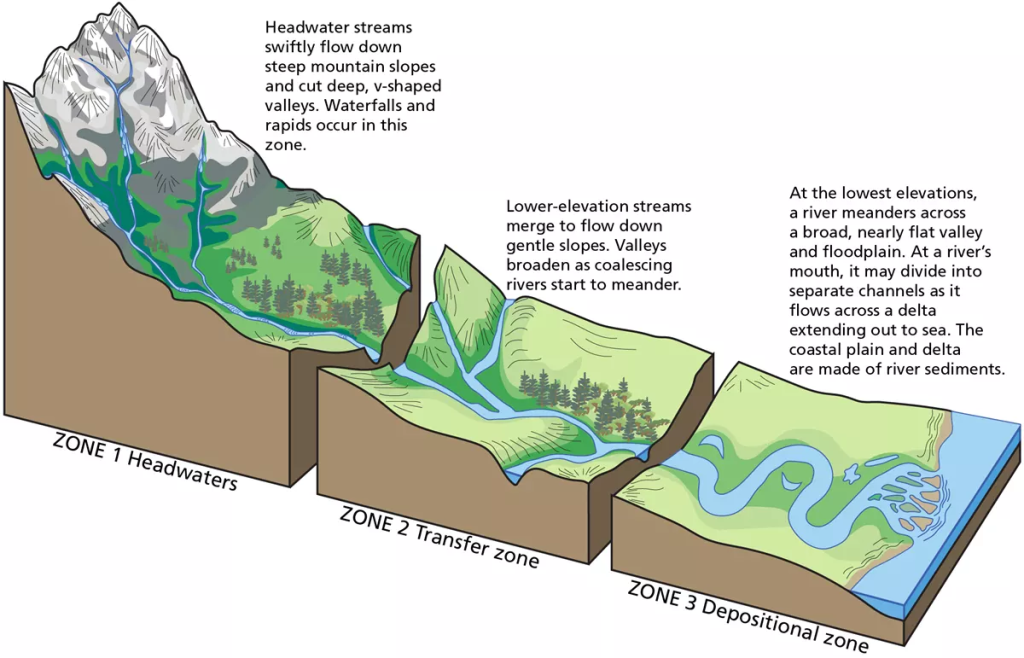
https://www.nps.gov/subjects/geology/images/3Zones_Fluvial_1204-2018_tte-01.jpg
In practice, many rivers show more complicated longitudinal profiles because of barriers to flow, such as resistant rock units, glaciers, or anthropogenic features such as dams. Such obstructions tend to form lakes which form local base levels along the course of the river. The longitudinal profile of the Colorado River in the USA shows a number of local base levels at lakes and dams.
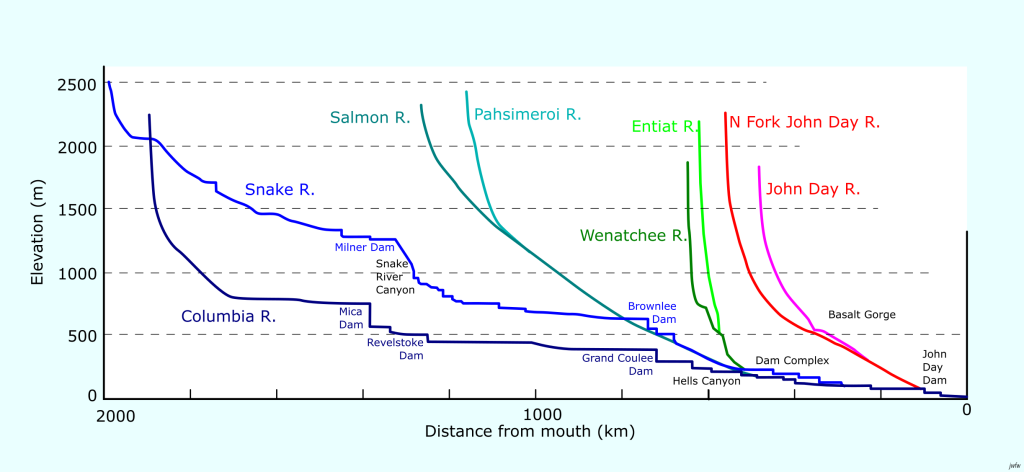
Over long periods of time (thousands to millions of years), rivers tend to smooth out such interruptions, by eroding obstacles and depositing sediment in lakes, restoring a graded profile.
The ability of a river to erode and transport solid material is strongly influenced by the slope. This is because the slope controls shear stress or traction the on the base and sides of the channel. Steeply sloping streams, typical of the headwaters of a river system, are described as having high energy[4] and are able to erode and move large particles as bed load. Gently sloping streams have lower energy and are less able to move coarse sediment, leading to more deposition in downstream portions of a river system.
In the following sections we will look at erosion and deposition in turn.
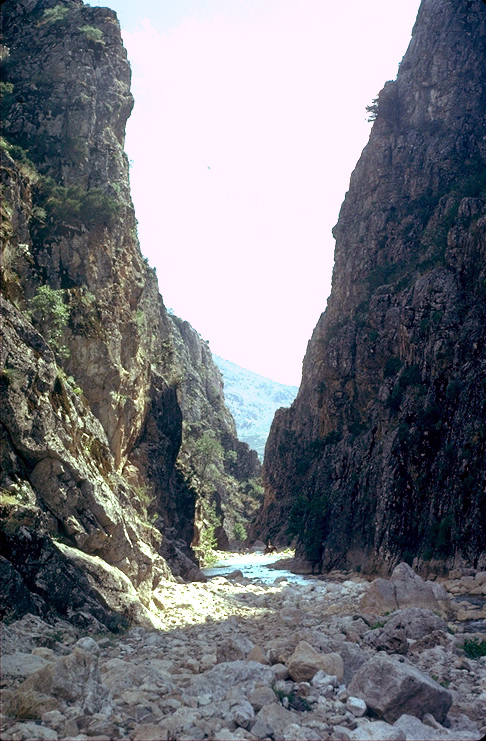
Erosion
Downcutting and valley generation
Erosion in the headwaters of a river system typically occurs at the base and sides of stream channels, leading to downcutting, the lowering of the floor of the channel as material is eroded. If the rocks are exceptionally strong, this may lead to the development of a slot canyon, a valley with almost vertical sides. More usually, however, steepening of the valley sides makes them unstable, and gravity brings material down the valley sides into the channel. Such gravity-driven processes are known as mass wasting.
Slope processes and mass wasting
Mass wasting includes a huge variety of processes[5], many of which are of major concern to human populations. The more rapid processes may cause death and destruction of property, whereas even slow processes of mass wasting can cause serious damage and destruction to buildings, highways, and other human infrastructure built on or near slopes. Civil engineers pay a lot of attention to mass wasting processes.
Rockfalls
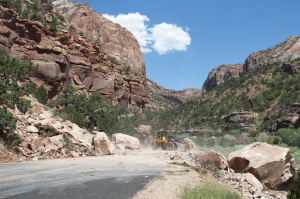
When rock falls from the steepest slopes, it moves downward through air under the action of gravity, either as single pieces, or as multiple fragments. Water is not involved in the mass transport process, although it typically plays a role in loosening the fragments before a fall.
Fallen rock material may accumulate at the base of a steep slope where it is known as scree or talus.
Slides
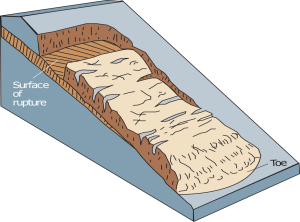
Coherent masses of rock or soil, that move above distinct failure surfaces, are known as slides. When the failure surface is approximately planar, the slide is known as a translational slide. Translational slides typically occur when there are planes of pre-existing weakness within the rocks, approximately parallel to the slope.
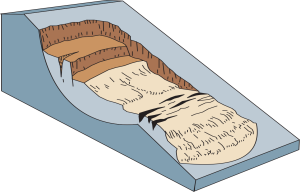
If the failure surface is curved, the slide is a rotational slide. Rotational slides can be recognized because the upper surface of the moved material is tilted inwards, toward the failure surface.
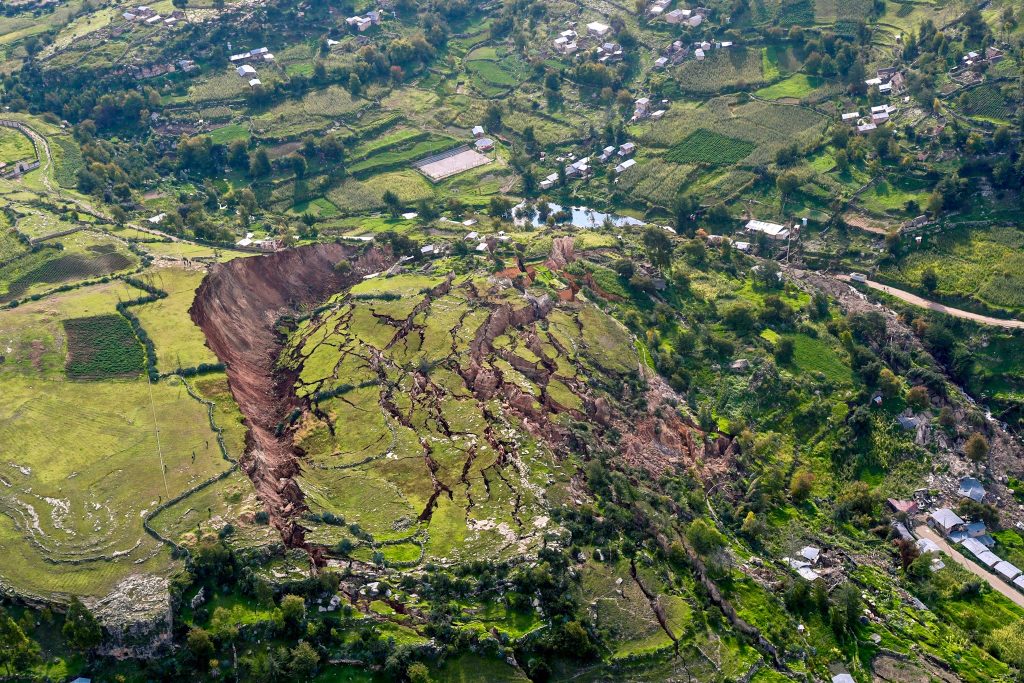
Flows
As material moves down-slope in a slide it may become progressively more disrupted. Such disrupted slides are sometimes called slumps although there is no sharp boundary between the two categories. With further disruption, the moving material may develop into a flow. Flows are masses in which the moving material loses coherence as it moves, behaving more like a liquid. Typically there must be either water or air present between the solid particles of rock, in order for a flow to move.
Debris flows
If moving material is water-saturated, it may develop into a debris flow, earth flow, or mudflow, in which all trace of the original structure is lost, and blocks of rock are carried along by a muddy matrix. Debris flows are some of the most hazardous mass-wasting phenomena as they may move fast (several tens of kilometres per hour) over large distances. Debris flows can carry blocks of rock that are metres or tens of metres across. Debris flows may be laminar (in which all the material is transported roughly parallel to the base of the flow) or turbulent (in which material is carried by swirling movements in the flow) like rivers, but the deposits of debris flows are typically very poorly sorted, containing a wide range of grain sizes.
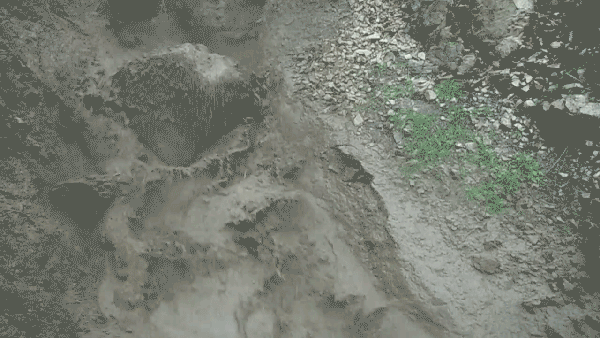
Debris flows are common on the slopes of volcanoes (covered in the section on igneous processes in the rock cycle) where they are known as Lahars.
Rock and debris avalanches
When a large volume of rock falls from a steep slope, collisions between fragments, and possibly the pressure of trapped air, may cause the falling debris to behave as a flow, which can continue moving on more gentle lower slopes. In some cases the runout of a debris avalanche may even extend some distance uphill on the opposite side of a valley, as happened in the case of the Frank Slide[6] in Alberta, Canada. In this event, a debris avalanche from Turtle Mountain obliterated part of the settler town of Frank, killing between 70 and 90 people and covering the floor of the Crowsnest river valley with debris, despite warnings from the Piikani Nation of the Blackfoot Confederacy. The events were described in the Canadian Mountain Assessment (2024) by Daniel Melting Tallow:
Piikani people have been in that area for thousands of years, and the Europeans came and found some coal in that area….The Piikani people were warning the people there: “Don’t live (there), don’t build your house. Build it further because that mountain is shaking” because they knew that. They didn’t listen to them: “Oh they’re just savages…” and stuff like that. They didn’t believe in their way of thinking and their knowledge and their knowing. Then they all settled in that area, and one night, the whole thing came down. The mountain came down and it buried a whole town. …..Underneath, the town is still there, and there are some bodies down there too. So our elders and our stories and our tales, they should be an addition to predicting what’s going to happen. Those Blackfoots knew what was going to happen… Canadian Mountain Assessment 2.6.1
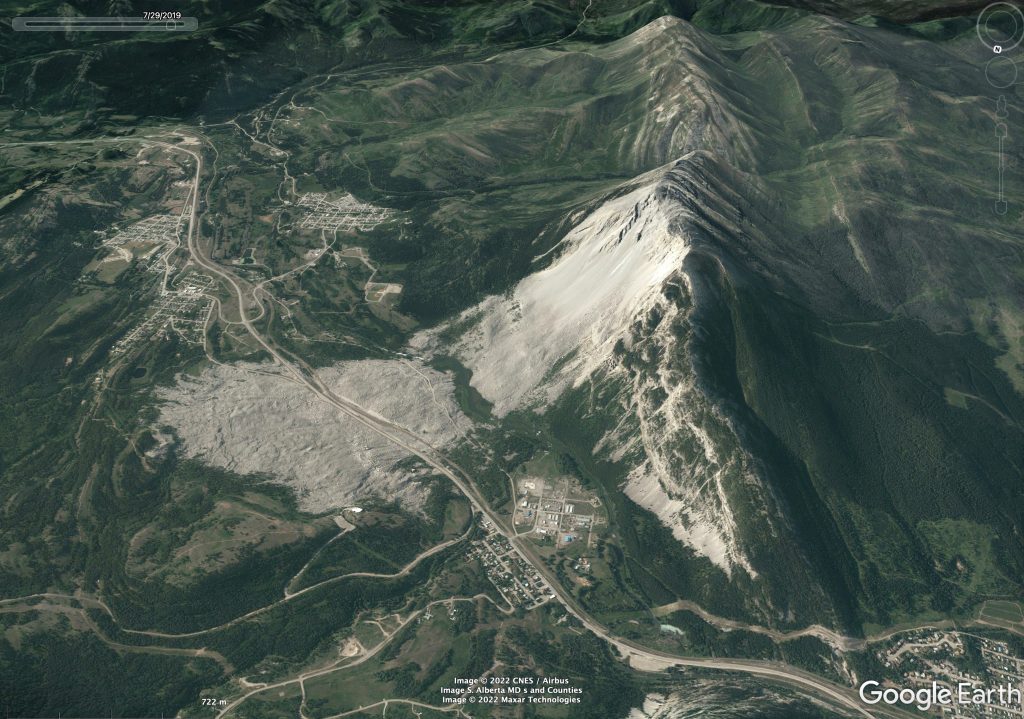
Creep
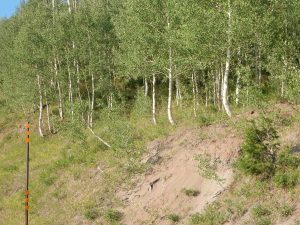
Slow movements, millimetres per day or less, occur on slopes, where they are promoted by changes in the amount of water in soil. Expansion and contraction occurs as soil is moistened and dried, and with each small movement, grains may shift slightly down slope. The resulting slow movement is called creep.
Creep is particularly effective in areas where groundwater is repeatedly frozen and thawed. As ice forms in the pore spaces, it expands and displaces the slope upwards and outwards slightly. When the ice melts, the grains settle more vertically downwards. This type of creep is called solifluction.
Over periods of years, these slow processes can move material large distances down slopes into valleys.
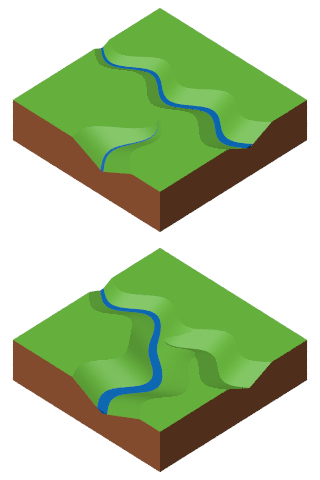
Headward erosion and stream piracy
Downcutting, combined with mass wasting, have unexpected consequences in the headwaters of some river systems. Rapidly downcutting streams oversteepen the region around their headwaters, causing their valleys to extend in an upstream direction, a phenomenon called headward erosion. Headward erosion may cause one stream to cut into another’s valley, eventually diverting the water in a process called river capture or river piracy. The result may be a large valley with little or no water in it, a telltale sign that river capture has occurred.
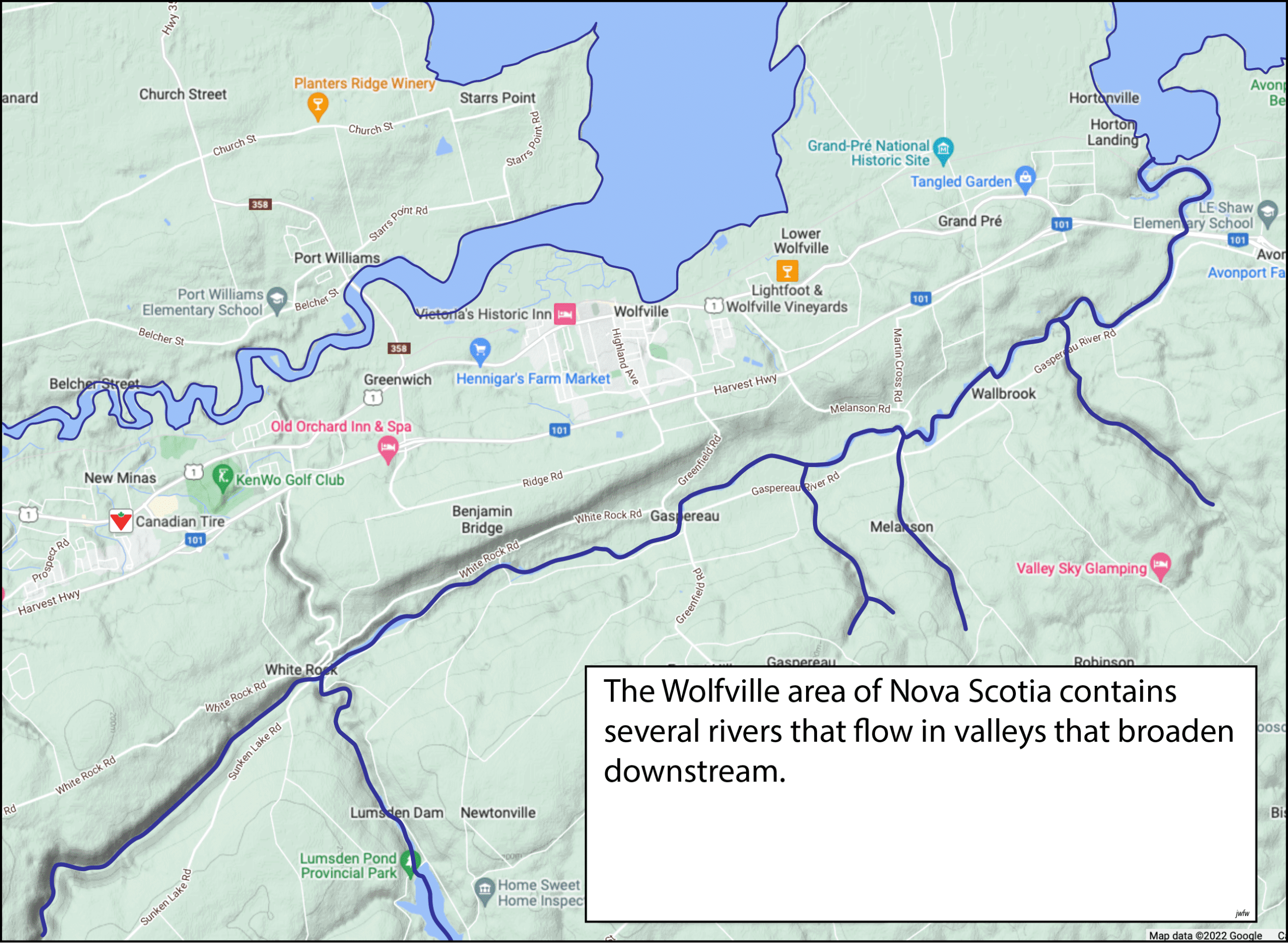
Lateral erosion
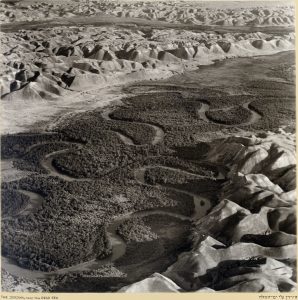
As streams approach their base level, downcutting becomes less and less effective. Rivers with low gradients carry out erosion by widening their valleys, rather than deepening them, a process called lateral erosion.Lateral erosion takes place particularly at bends in the course of rivers. Because of the behaviour of water in channels (see above), even a small bend in a river tends to be amplified over time, because the outer bank is eroded fastest. This process is an example of positive feedback — whereby a small change in a system leads to a process that amplifies that change. Lateral erosion gradually pushes out the sides of a valley, creating a broad floodplain in which both erosion and deposition may occur.
Depositional landforms
In the downstream parts of river systems, the velocity of water near the river bed decreases, and rivers become less able to transport sediment, which may be deposited in a variety of depositional landforms.
Alluvial fans
When a mountain stream emerges onto a broader valley floor, the velocity of flow may decrease abruptly, leading to the dumping of large amounts of sediment in an alluvial fan. In longitudinal river profiles, alluvial fans typically occur at a break in slope, and the deposition of the fan tends to smooth out the profile so that it more nearly approaches a graded profile. In an alluvial fan, the main channel of a river branches in a downstream direction, forming multiple distributariesAlluvial fans have a similar geometry to deltas. In both cases a river branches into multiple distributaries. Both are formed when flow in a river channel is abruptly slowed down. Alluvial fans form when that slowdown is due to a break in slope whereas deltas form where a river enters standing water — the sea or a lake. If a steeply flowing stream directly enters a standing body of water, the two process are combined and the result is called a fan delta. Typical sediments in alluvial fans are gravel and coarse sand.
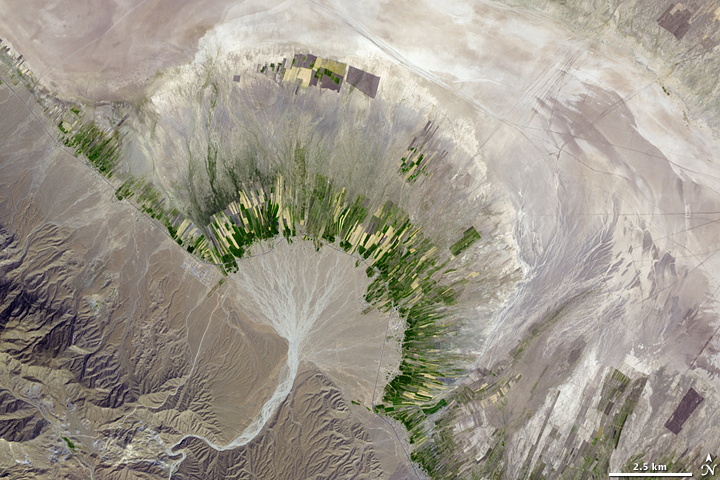
Fluvial deposits
The sediments deposited by rivers are described as fluvial. River systems that deposit sediment show enormous variety, but two end-member types of geometry are frequently seen: braided and meandering. Note that not all rivers can be slotted in to one of these categories; there are many intermediate types of rivers.
Braided systems
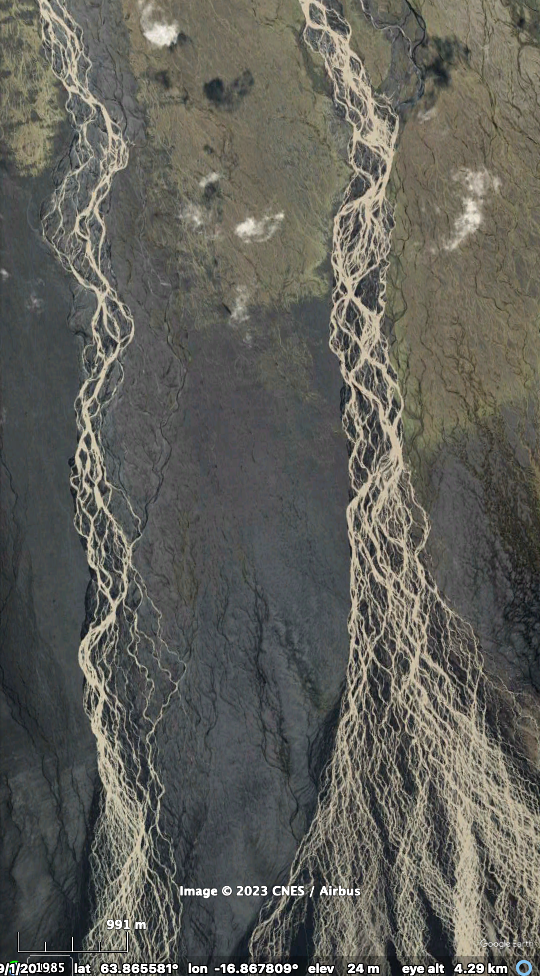
Braided river systems have multiple channels that branch and rejoin repeatedly downstream. Deposition takes place predominantly in bars that are located between the channels. During low discharge periods, flow is confined to the channels, but during flood stages the river covers the bars and deposits sediment on top of them too. Braiding in rivers seems to be encouraged by a very episodic flow, high bed load, and lack of vegetation. Braided rivers are common around glaciers, where summer melting supplies large volumes of sediment, and in arid areas with little vegetation.
Meandering systems
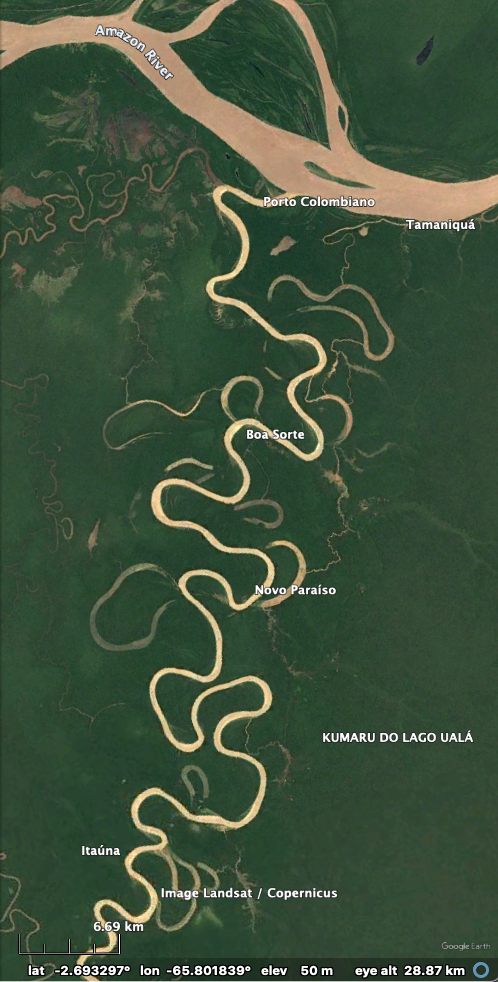
Meandering river systems typically have a single channel active at any one time, but lateral erosion causes that channel to migrate, sometimes spectacularly, across a broad valley. The portions of the valley floor on either side of the channel make up the floodplain.
The behaviour of meandering channels is explained by a process of positive feedback whereby a small bend focusses the fastest flow, and therefore the most rapid erosion, on the outside of the curve, which in turn enhances the bend. Deposition, typically of sand, occurs on the inside of the curve where the current is slower, producing a point-bar. Bends which cause a large change in direction (more than about 90°) are called meanders.
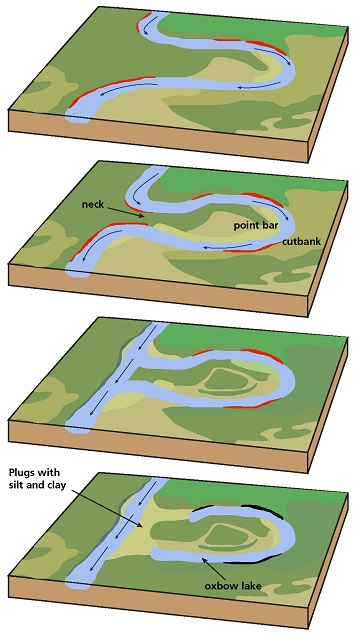
Meander development does not continue indefinitely. Eventually a narrow meander neck develops, which may be eroded during a high discharge event, straightening the stream. The isolated meander channel then forms an ox-bow lake.
Floodplains
A flood occurs during high-discharge events, when a river’s discharge exceeds the capacity of its channel. The river spills out of the and expands over its floodplain. The rate of flow drops abruptly as water leaves the channel, and the fine-grained suspended load of the water is typically deposited across the floodplain. Floodplains are typically very fertile: in natural environments they have dense growth of plants. Humans exploit floodplains for agriculture and habitation; river channels are used by humans for transportation of goods. It's likely that plants contribute to the stabilization of river banks, thereby encouraging the development of meandering, rather than braided, rivers. Prior to the evolution of abundant land plants around 400 Ga, it appears that meandering rivers were less common.
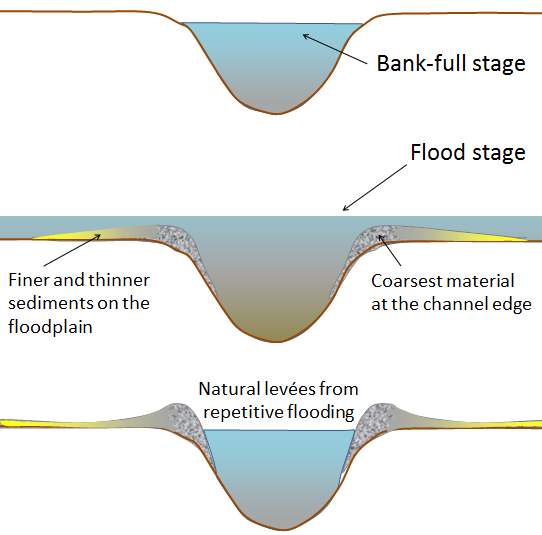
Rapid deposition at the edge of a channel may deposit a raised bank, called a natural levee (French levée). Levees are responsible for negative feedback, because they hinder further flooding. Humans living on floodplains have typically exploited levees by reinforcing them and raising them higher to prevent flooding. However, under natural conditions, periodic flooding events distribute fine-grained sediment across floodplains, gradually raising them and contributing to their agricultural fertility. Construction of artificial levees locally reduces the frequency of flooding, and delivers more sediment downstream, where it may lead to new problems including obstruction of channels used for navigation, and increased flooding risk.
Flood risk may be assessed by examining hydrographs over decades or centuries, if data are available. A recurrence interval is the average period of time between two floods of the same magnitude. These intervals are determined by plotting the frequency of past floods using data gathered from the gauging stations over decades or longer. Sometimes, it may be possible to collect data from floodplain sediments that also help to determine flood risk.
Particular sizes of flood are characterized by their recurrence interval. For, example, a 50-year flood is one of a size that recurs on average every 50 years. In other words, there is a 2% chance that such a flood will occur in any given year. Note that because of the unpredictability of discharge, these estimates are averages. The actual intervals beteween "50-year" floods may be much shorter, or much longer.

River mouths
When a river enters the sea, the velocity of channel flow decreases further, and flow may be modified by the action of waves and tides. Distinct depositional landforms called deltas and estuaries are developed. Because of the importance of the sea to their formation, these features are discussed in the chapter on seas and oceans.
Effects of base-level changes
Rivers erode laterally and by downcutting. Downcutting occurs particularly when sea-level falls (base level change), when the land surface is lifted (for example when isostatic rebound occurs following the melting of glaciers), when an obstacle is removed, or when discharge increases. Conversely, if sea-level rises, the land subsides, discharge decreases, or when a river is blocked by an obstacle, then sedimentation and/or lateral erosion are favoured. Changes in the history of a river system may leave their mark in the landscape.
Terraces
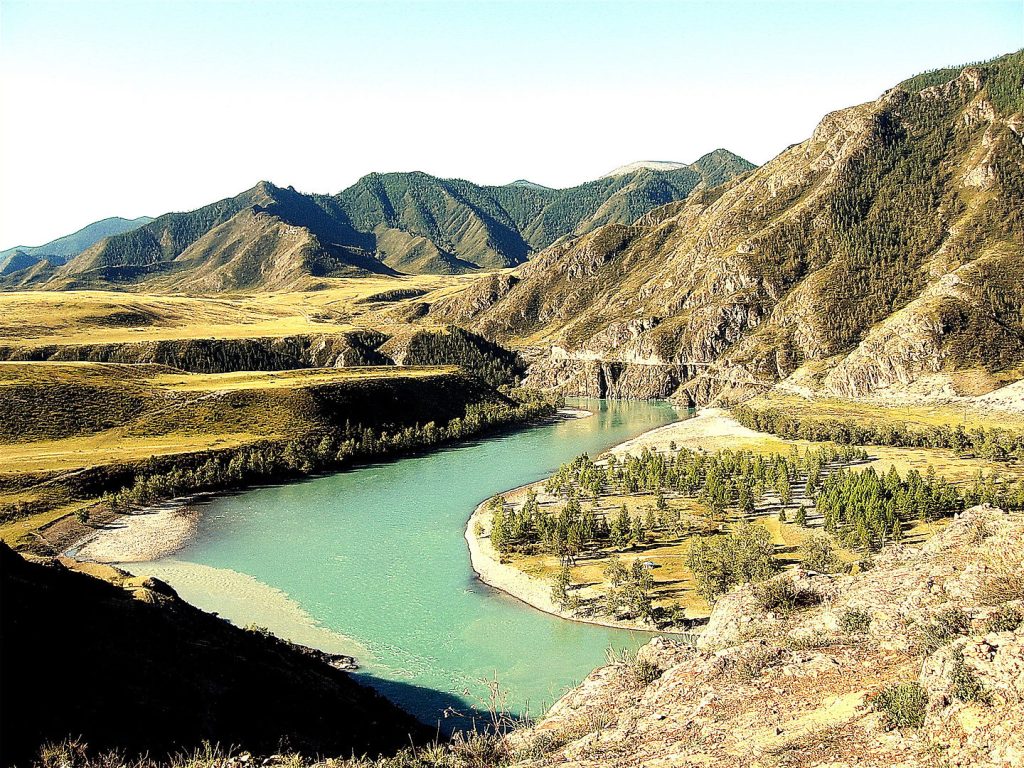
Rivers that have been affected by repeated periods periods of lateral erosion or sedimentation that have alternated with of downcutting show valleys with river terraces: flat areas part-way up the valley sides. Each terrace represents a pause in downcutting when the river has widenened its valley and floodplain. Renewed downcutting leaves parts of the abandoned floodplain on the valley sides.
Incised meanders
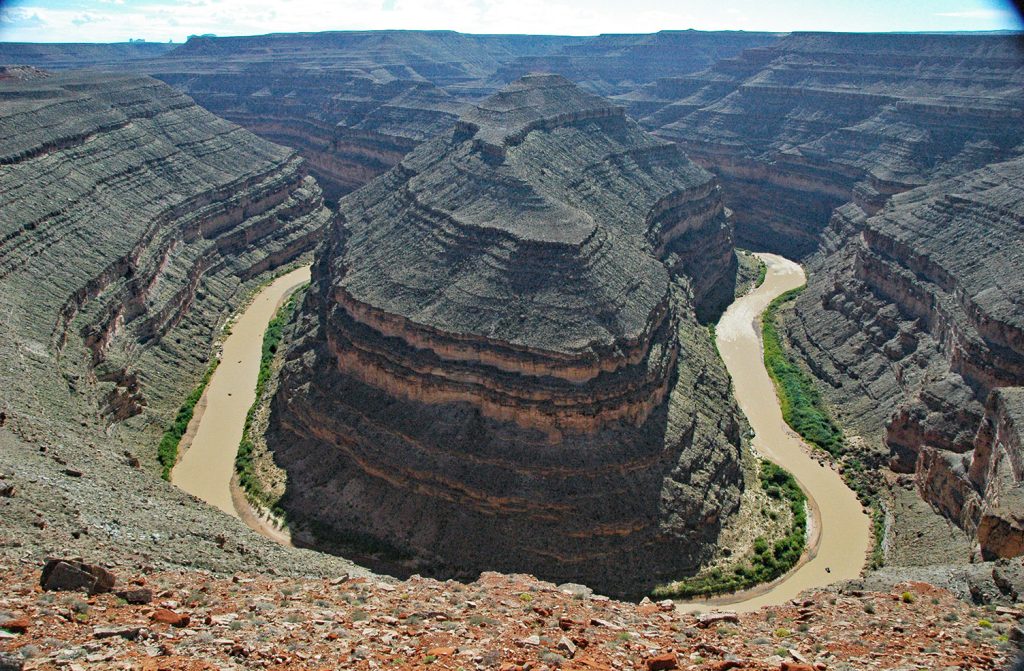
When the base-level of a river falls dramatically relative to the valley floor, a river that has evolved into a laterally-eroding, meandering system may suddenly start downcutting again. Meanders that existed in the previous life of the river may become incised into the landscape by downcutting. In this way, meandering rivers may be preserved at the bottom of steep-sided or terraced valleys.
Superimposed drainage
The process of incision may produce a river channel that appears to have no relation to the bedrock features it flows through. For example, the Susquehanna River in Pennsylvania, U.S.A., shows a gently meandering shape typical of mature low-gradient rivers in broad floodplains, yet it cuts right through the "valley and ridge" province of the Appalachian mountains, formed of tilted and folded layers of erosion-resistant rock. The channel was presumably developed when the river flowed in a floodplain developed on flat-lying layers of younger rock like the present-day Mississippi system. Those younger layers have been removed by erosion, and downcutting has preserved a channel shape that is unrelated to the rocks through which it flows. Some smaller, younger tributaries of the Susquehanna do follow the geological structure; they presumably developed after the downcutting process.
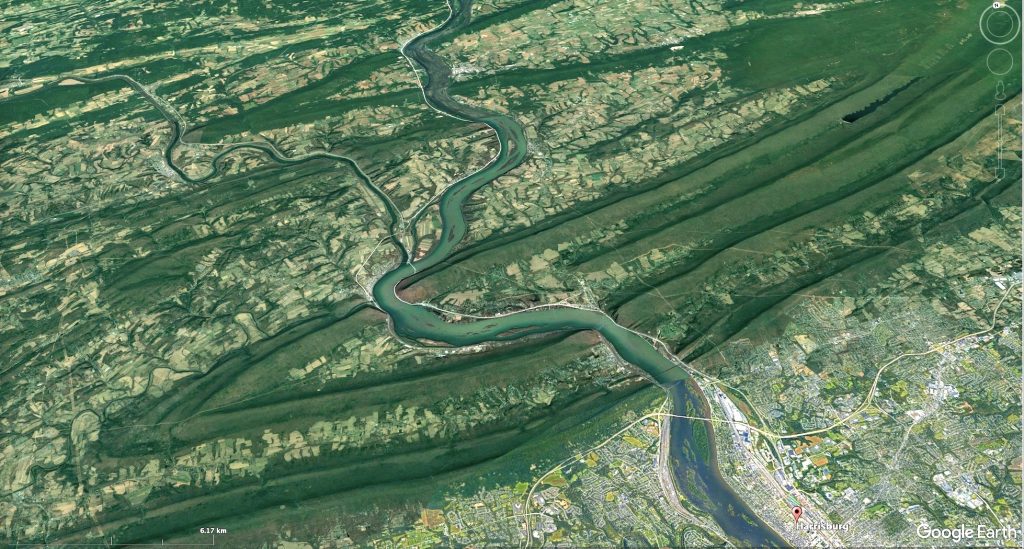
Lakes
A lake is a standing body of water filling a depression on land.
Origin of lakes
Some sort of obstacle to flow is typically present to help produce a lake. For example:
- Glaciers leave many erosional and depositional features that can obstruct water flow;
- Volcanic activity forms craters and blocks rivers with lava dams;
- Tectonic movements produce faults and deep rift valleys that may trap water in lakes;
- Humans erect dams to provide irrigation water and generate power.
Lakes produced by any of these process may form local base levels in longitudinal river profiles.
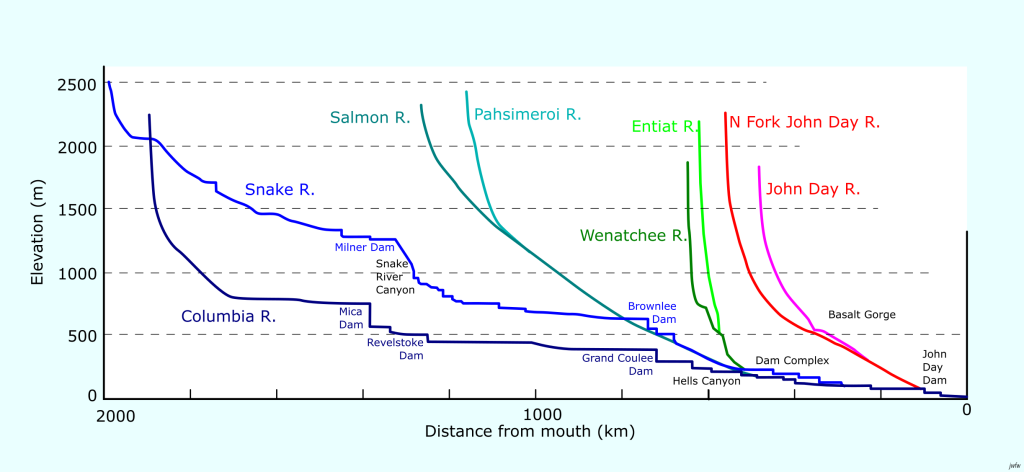
In addition:
- Rivers themselves may form lakes as part of their normal evolution, for example by cutting off meander channels leaving ox-bow lakes.
Most lakes are short-lived on geological time scales. The obstruction that produced a lake may be eroded away, while sediment and organic matter may accumulate in the lake, eventually filling them in. Some lakes transition over time into to wetlands. Some may evaporate away.
An importand variable that controls the behaviour of lakes over time is the nature of any outlet: the place where water leaves the lake. This characteristic is used to classify lakes into open and closed categories.
Open lakes
Open lakes are those which have an outlet with discharge comparable to the inflow that provides water to the lake. Typically the outlet is a stream, where the lake spills over a barrier into a river. Some open lakes have underground outlets into cave systems. Generally, their lake levels do not fluctuate much because when inflow increases, outflow also increases.
Because river water slows down dramatically as it enters a lake, coarse, bed-load sediment tends to be deposited near the lake shore. Fine grained muds settle out from the suspended load in offshore parts of the lake. The dissolved load travels mainly through the lake into the outlet, and most open lakes have relatively fresh water.
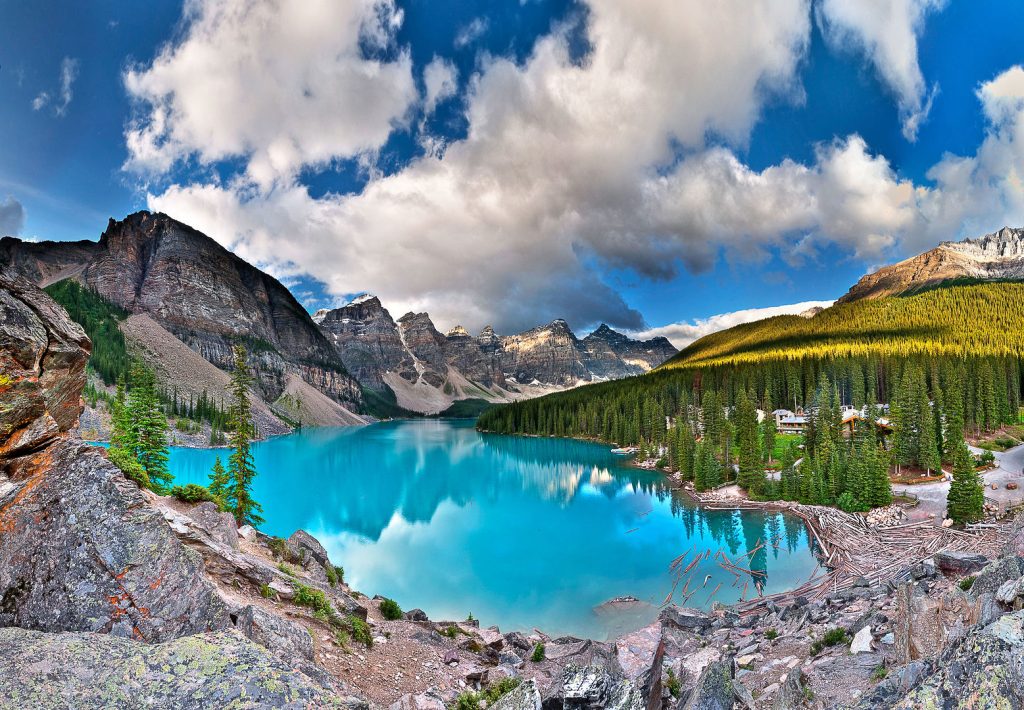
Closed lakes
Closed lakes have an inlet stream, but do not have an outlet stream. They tend to form in arid climates — places where evaporation is greater than precipitation. The water that enters the lake can only leave through evaporation, so dissolved ions derived from the dissolved load of the incoming river accumulate, and the lake becomes saline or salty. Water level fluctuates a great deal in most closed lakes, as a result of the changing balance between preciptiation and evaporation.
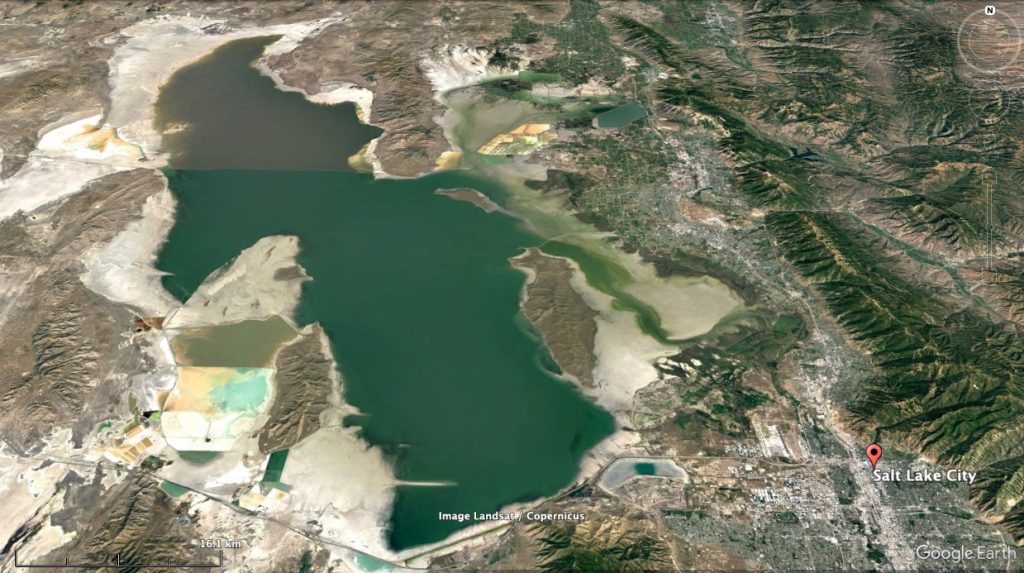
The sediments formed in closed lakes typically include salt deposits formed by evaporation, in addition to the sands and muds also typical of open lakes.
- This statement neglects a small amount of interflow: water that travels in the vadose zone between the land surface and the water table. However, the rate of flow in the vadose zone is typically low. Groundwater concepts are explored more fully in another section. ↵
- To do this conversion, remember that the number of cubic metres in a cubic kilometre is 1000 × 1000 × 1000 or one billion, 109 m3. So, to convert from cubic kilometres per year to metres per second we must multiply by 109 and then divide by the number of seconds in a year, about 31.5 million. ↵
- A note on the term "watershed": In British usage the term "watershed" means "drainage divide", typically a ridge from which water is "shed" to either side. However, in North America "watershed" is commonly used to describe a whole drainage basin, an area bounded by drainage divides. Because of this ambiguity we avoid the term "watershed" in the main text. ↵
- Strictly speaking, we should say "high power" because we are talking about the rate at which potential energy of the water is converted into mechanical energy of movement. However, the term "high energy environment" is most used in the study of sedimentation. ↵
- Many of these processes are termed "landslides", but this term is used in several different senses. For some, it can include almost any mass wasting process, but others include only those processes where a distinct slide surface is present. Because of this ambiguity we avoid the term "landslide" here, but we do follow the general classification of mass wasting phenomena laid out in Highland & Bobrowsky (2008) "The Landslide Handbook" US Geological Survey Circular 1325) ↵
- The name "Frank Slide" is used for this event and its deposit, for example in local museums, memorials, and guidebooks, but by most definitions it was not a slide but a flow. ↵
The removal of material from a site of weathering on the Earth's surface.
Flow of water in a sheet over the land surface, without channel formation
The movement of water through confined channels over Earth’s surface.
Water that occupies spaces within the Geosphere
Water transported into a channel through groundwater flow into the base of the stream
The force that operates between a flowing liquid and an adjacent solid
A measure of the resistance of a fluid to shearing; measured as the force concentration needed to achieve a given velocity gradient. In everyday terms, a measure of hhow 'thick' a fluid is.
Having a large number of bends (e.g. of a river channel)
(1) The total rate of flow passing through a channel, calculated as a volume per unit time;
(2) Any exit of water from groundwater onto the Earth's surface.
Graphs of river discharge over extended periods of time, indicating the rise and fall of total flow.
Smaller streams that flow and converge downstream into a larger channel.
The total area that delivers water into a single channel or waterbody.
A border between drainage basins.
The rate of change (with distance) of velocity in a fluid such as water
The deviation of a surface from horizontal; may be measured as an angle or as a tangent (rise or fall divided by horizontal "run").
A plot of elevation versus downstream distance in a river.
The elevation at which a river enters the sea or a lake
Generated by humans
The elevation of a river at a point where the speed of flow is near zero, typically at a lake.
Force per unit area acting parallel to a surface
Force per unit area; also known as stress
The erosion of the base of a steeply sloping stream, particularly near the headwaters of a river.
A valley with exceedingly steep sides, created when a channel downcuts through strong rocks.
Processes that move dense solid material down slopes on the surface of the Geosphere due to the force of gravity.
Fallen rock material that accumulates at the base of a steep slope.
Scree; fallen rock materail accumulated at the base of a cliff or other steep slope
Mass downslope of coherent material along a basal failure surface
The movement of a rock mass down slope along a planar failure surface.
The movement of a rock mass down slope along a curved failure surface.
Slides in which the moving mass is significantly deformed; transitional into flows.
Any movement of material down a slope in which the material behaves as a fluid, deforming continuously as it moves.
Mass transport in which large fragments are supported by the strength of a wet mud matrix that flows
.
A debris flow on the land surface
A flow of fine-grained sediment mixed with water
Sheet-like; applied to flow of fluids, it describes flow along sub-parallel lines, lacking the gyres typical of turblence.
Having turbulence: wirling movements in a fluid.
Having a very wide range of grain sizes
A debris flow generated by volcanic activity
The extent of a flow beyond the slope on which it originated
Creep observed when groundwater freezes and thaws, allowing sediment grains to move down the slide at an annual rate.
The extension of the head of a river valley in an upstream direction, resulting from downcutting, coupled with downslope movement of material.
The diversion of water from one stream into another channel, as a result of progressive river erosion (usually headward erosion).
The widening of a river valley by a low-gradient river. This process typically occurs when the bends of rivers reach the valley sides.
A cycle in which the products of a process act as inputs increasing the activity of the same process
The land surrounding a river channel which becomes submerged during high-discharge events (floods).
A seimi-circular or triangular body of coarse sediment formed at a break in slope in a river system
Channels that branch from a main channel in the downstream direction, typical of alluvial fans and deltas
Pertaining to rivers.
Braided streams have multiple branches that branch and rejoin
River behaviour characterized by large changes in channel direction (bends).
A depositional landform, typically of sand or gravel, in a river
The inner edge of a river’s meander, where sediment can accumulate due to the slower flow of water.
A lake formed when a meander channel is abandoned during hte evolution of a river.
Fine-grained sediments that may be suspended within turbulent waters.
A raised bank along the edge of a river channel due to deposition during flooding.
A cycle in which the products of a process act as inputs inhibiting the same process, reducing the effects of the process.
Structures build by humans along the banks of rivers, with the purpose of preventing or reducing flooding.
The average time between successive floods greater than or equal to a specified magnitude.
A sediment deposit formed from the flow of a channel into a standing water body.
A partially enclosed bay or river mouth in which freshwater and seawater mix
Flat areas on the sides of a river valley, and elevated above present-day floodplain level. Terraces are formed when renewed downcutting carves through a former floodplain.
A body of surface water that is isolated from the world's oceans.
Lakes with an outlet, such a stream, with discharge equal to that of the lake’s input; open lakes typically contain fresh water.
A lakes that receives input, yet lacks an outflow other than evaporation.
Sediment carried along the water-bottom (usually of a river) through rolling, sliding, and saltation
Material that travels in flowing water in solution, rather than as solid particles
Containing a high concentration of dissolved salts