4 The Atmosphere: What is it Made of?
This chapter is a pre-review version
Learning Outcomes
By the end of this chapter you should be able to:
- Explain and distinguish between heat, temperature, and pressure in gases;
- Describe the principal layers of the Earth’s Atmosphere;
- List the main components of the Earth’s Atmosphere;
- Explain how water condenses as air cools.
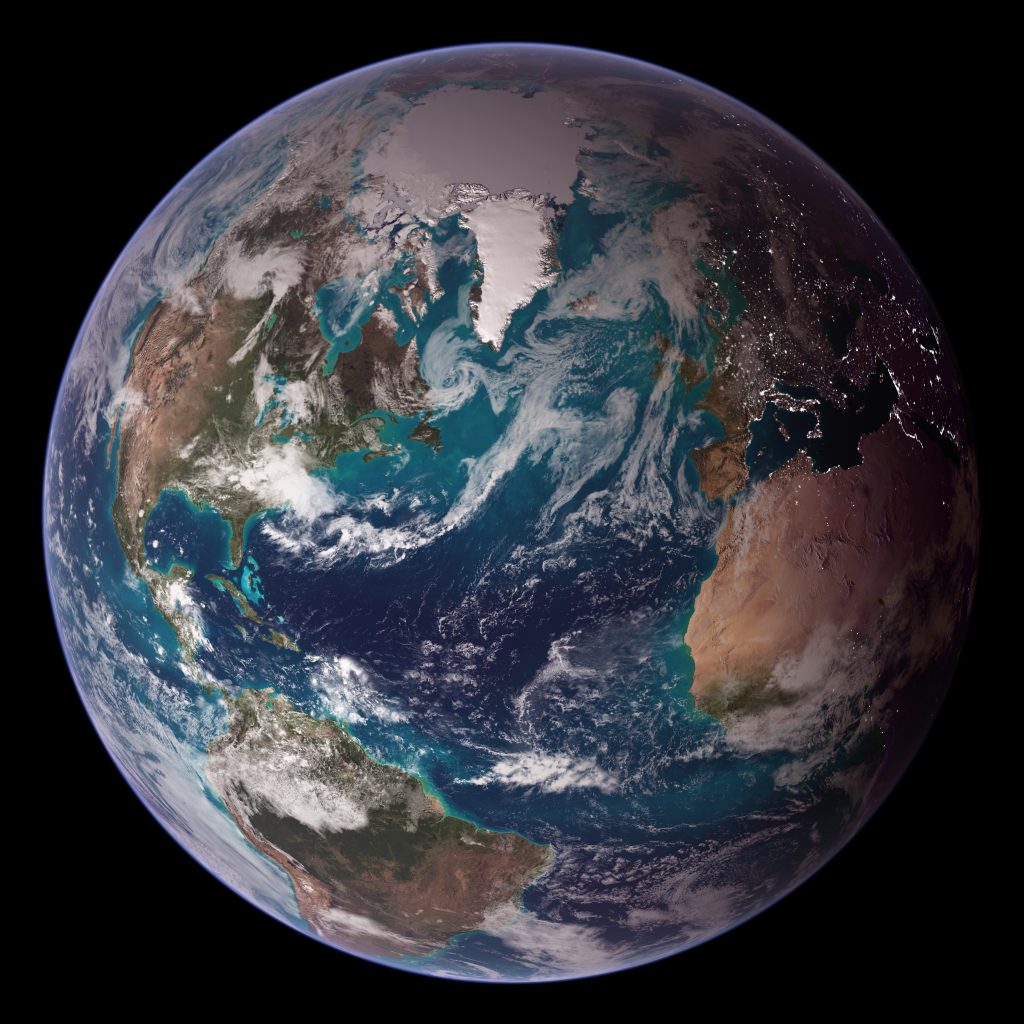
The outer parts of most of the planets in the Solar System are made mainly of gases; this gaseous outer part of a planet is called its atmosphere. For us, Earth’s Atmosphere is all important – it provides the oxygen we breath, it controls the weather and climate that we experience, and it provides water to the land surface, in the form of rain and snow, on which all land plants and animals depend.
When we look at images of the planets in the Solar System, sometimes the disk we see is actually the atmosphere. This is true of Venus, and the “gas giant” or Jovian planets Jupiter, Saturn, Uranus, and Neptune. The atmosphere of Mars is quite transparent, so we see the rocky surface of the planet. Mercury, like Earth’s Moon, has almost no atmosphere, so images show the rocky surface too. The Earth is special: views of the Earth from space show white, reflective clouds, but with enough clear areas that the surfaces of the land and oceans are also visible. Those cloud patterns reveal a constantly changing Atmosphere. As inhabitants of the Earth we experience those changes as weather.
Earth’s Atmosphere extends above the land and ocean surface and fades into space between ~100 and 1000 km higher, depending on the criteria used. The outer edge of the Earth’s Atmosphere is quite hard to define, as it fades away into the emptiness of space. One suggestion is known as the Kármán Line, and is set at 100 km. For legal purposes, it’s the upper limit of national airspace.
The Atmosphere supports and protects life, stores moisture and solar energy, and moves materials around in the Hydrosphere and on the surface of the Geosphere. The Atmosphere, especially the lowest part, called the troposphere, constantly changes. Short-term changes, from seconds to weeks, result in weather, studied by meteorologists. Longer-term characteristics of the Atmosphere, from a few years to billions of years, are described as climate, studied by climatologists (or climate scientists). Studies of climate must use averaged weather conditions. 30 years is a common time period for calculating climate averages.
Gases: temperature and pressure
The Earth’s Atmosphere is largely composed gases:— chemical substances in which the molecules are in constant motion, colliding with one another, and against the walls of any solid objects in the Atmosphere. The Atmosphere is a mixture of gases called air. The most abundant gas in the Atmosphere is nitrogen, but of course oxygen is the most important to animal life, including humans.
Unlike atoms and molecules in liquids and solids, the molecules of gases are not connected to one another by any kind of atomic bond. The molecules in a gas are in constant motion, and the average kinetic energy (energy of movement) of the molecules in a gas is measured by its temperature. In everyday life, temperature is measured above and below an arbitrary zero point (0°C in the Celsius scale). However, scientific temperatures are measured in kelvin (K). The zero on the kelvin scale is the point where molecules have no kinetic energy, known as absolute zero. Zero kelvin is about -273°C.
The temperature of a gas is not the same thing as its heat. Heat measures the total kinetic energy of the molecules in a mass of gas. In scientific terms this is expressed by saying that temperature is an intensive variable whereas heat energy is an extensive variable. What this means that if measure the amount of heat in an amount of gas, and then double the amount of gas, we have double the amount of energy. However, the temperature does not change when we double the quantity. In the upper Atmosphere the air is very thin, so there are not many molecules, but the average kinetic energy of each one is high. There, the temperature may be very high but the amount of heat energy is quite low.
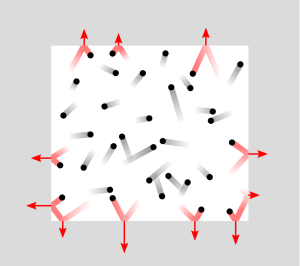
The motion of gas molecules causes impacts against any solid object, and that results in a force. The pressure is the force that acts on a unit area (e.g. a square metre) of any solid or liquid surface that a gas is in contact with.
The pressure of a gas is closely related to its volume: if you squeeze a gas into half its volume, without changing the temperature, the pressure doubles (a relationship known as Boyle’s law). Conversely, if you reduce the pressure (for example by raising a mass of gas from sea level to a position high in the Atmosphere) it expands, and the density (mass per unit volume) decreases.
In the Atmosphere, the pressure and density are lowest at the edges of space, at the top of the Atmosphere over 100 km above the surface of the Geosphere. The weight of this gas presses on parts of the atmosphere below. Each lower layer of the Atmosphere is compressed by the weight of the air above it, with the result that the pressure and the density of the Atmosphere increase downward.
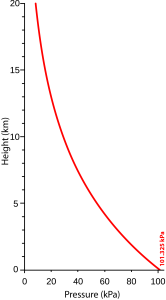
The average pressure of the Atmosphere at sea level is about 101325 Pa or 101 kPa (kilopascals). This pressure is sometimes referred to as 1 atmosphere (atm).
101 kPa = 1 atm
As you might expect, there is a relationship between the temperature and the pressure of a gas, because both are caused by the motion of the molecules. If a gas is heated inside a sealed container, the pressure rises in proportion to the absolute temperature (in kelvin). Eventually this may cause the container to explode. (Don’t try this at home!) On the other hand if a mass of gas is heated in the Atmosphere, not in a container, it is free to expand and its density falls. This has several important consequences. First, hot air will tend to rise in the Atmosphere, and cold air will flow in to take its place. Second, where solar heating is most intense, near the Equator, the pressure falls because the density of the overlying Atmosphere is less.
The behaviour of rising or falling air depends on whether heat is added or not. If no heat is added or removed, the movement is described as adiabatic. If no heat is added or removed, you might think that the temperature would stay the same, but actually this is not the case. This is easily demonstrated by pumping up a bicycle tire. As you work the pump, you transfer mechanical energy to the air as you squeeze it into a smaller space. That energy is converted to heat, and the temperature rises. As air rises in the Atmosphere, the opposite happens. The air expands, and this means that work is done against the pressure of the surrounding air, so some energy is lost in the form of mechanical work. As a result, rising air cools. The rate of cooling is known as the adiabatic lapse rate. For dry air the lapse rate is 9.8°C per kilometre, or about 1°C per hundred metres. This is one of the reasons air is typically cooler on the top of a mountain than it is in an adjacent valley. The lapse rate for damp air may be different, but before considering the behaviour of damp air, we need to look at the variation of temperature in the Atmosphere.
Vertical changes in the Atmosphere
The temperature in the Atmosphere changes in quite a complicated way, as shown in the diagram below. To understand how this works, we need to consider where the energy is coming from.
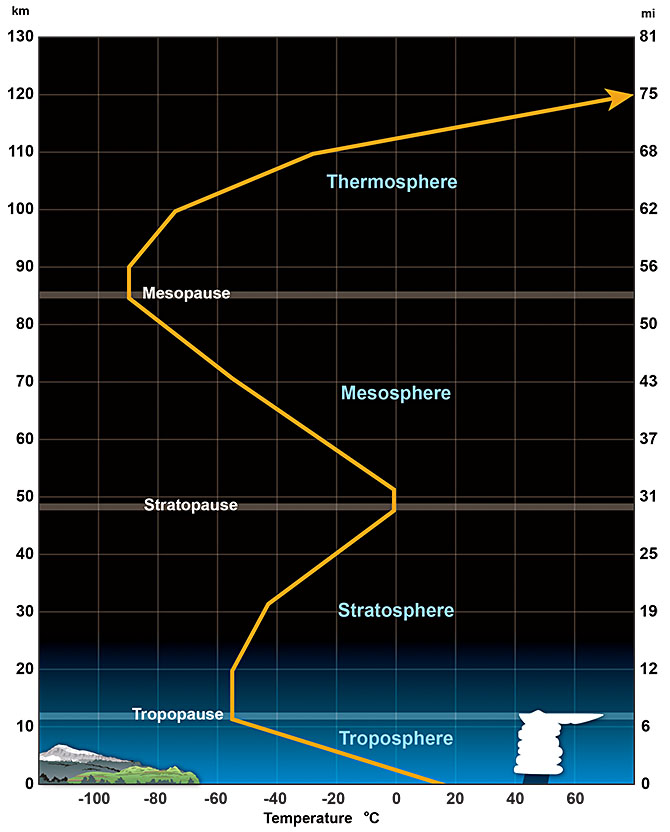
The outer parts of the Atmosphere are very thin. Although there’s not much energy in it, the molecules are so dispersed that each one has quite a large amount of kinetic energy so the temperature is high. Outer regions of the atmosphere are known as the thermosphere. As the air becomes more concentrated downwards, the amount of heat energy per molecule declines so the temperature falls to a minimum around 80 km above the Earth’s surface, known as the mesopause. Below it is the mesosphere. In both regions, solar radiation removes electrons from atoms and molecules, producing charged ions: the mesosphere and thermosphere are parts of the larger ionosphere. Surges of charged particles from the sun (solar wind) may change the ionization state of atoms and molecules, particularly in the thermosphere, producing visible light – the aurora borealis (northern lights) and aurora australis (southern lights).
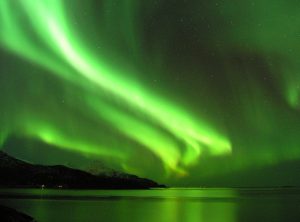
Around 50 km, oxygen and ozone in the Atmosphere become concentrated enough to start absorbing large amounts of incoming radiation, especially in the ultraviolet range. This trapped energy heats the air, so there is a temperature maximum at about 50 km, known as the stratopause. The temperature at the stratopause may be similar to that at the Earth’s surface, and this heats the mesosphere from below, accounting for the downward rise in temperature. The stratopause marks the top of the stratosphere. The temperature in the stratosphere increases upward, because it is warmed by ultraviolet absorption around the stratopause.
Continuing downward, the next big absorber of solar radiation is the land or ocean surface, which is intensely warmed by solar energy. Therefore, the bottom part of the Atmosphere, known as the troposphere, is heated from below. Temperature decreases upward to a boundary about 10–15 km above the land or ocean surface, known as the tropopause, marking the transition from stratosphere above to troposphere below. The height of the tropopause, and the thickness of the troposphere, is actually quite variable. Near the Equator, where the ground is intensely heated, the troposphere may extend up to about 17 km. In polar latitudes, with less solar heading from below, the troposphere is typically less than 10 km thick. Almost all weather happens in the troposphere.
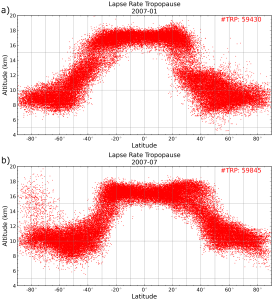
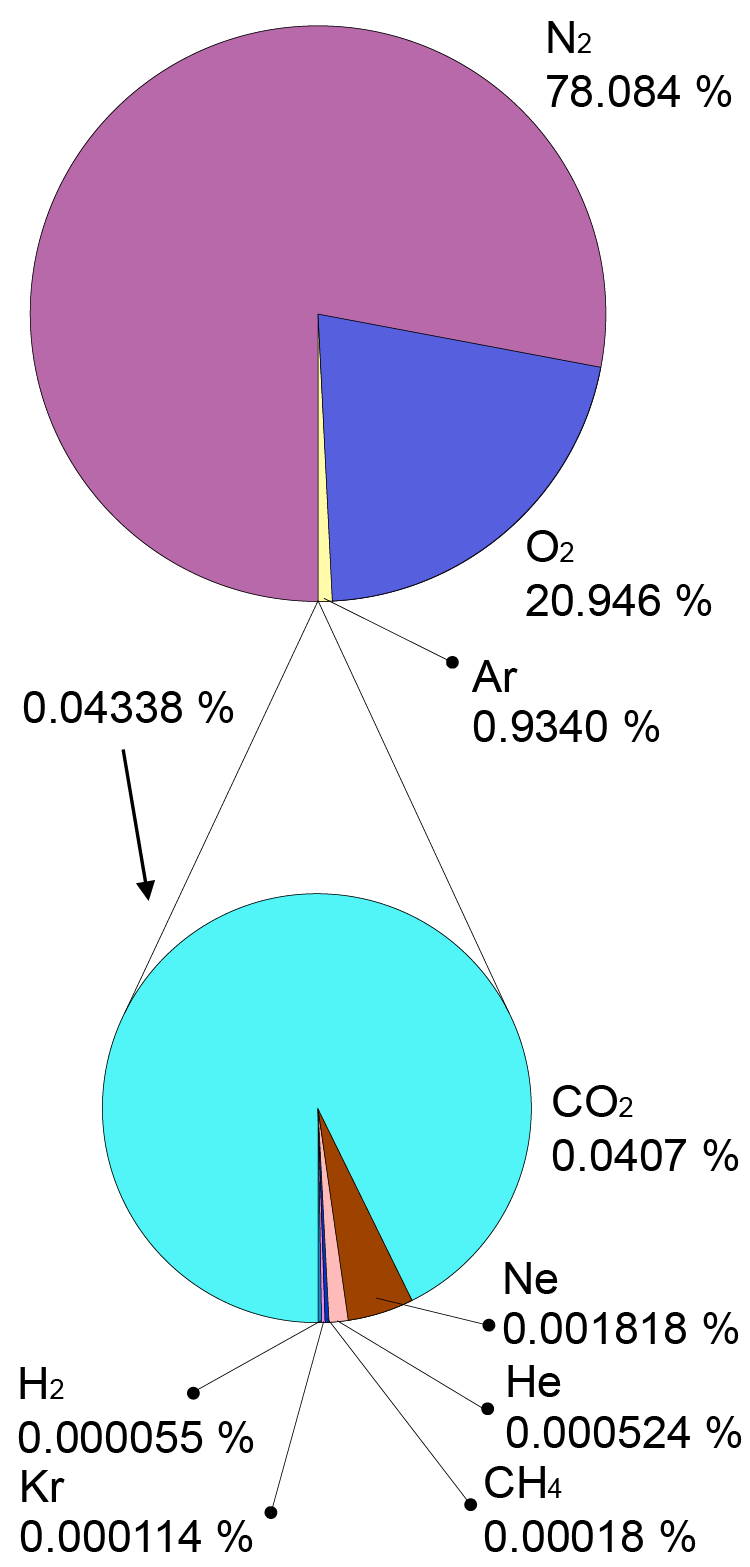
Composition of the Earth’s atmosphere
Mixed gases: partial pressure
The atmosphere is a mixture of different gases, as we shall see below. In a mixture of gases, it’s possible to break down the total pressure into partial pressures exerted by the different gases. Dalton’s law of partial pressures states that the total pressure of the mixed gas is the sum of the partial pressures of each gas in the mixture. The partial pressure of each gas depends on the proportion of its molecules in the mixture. For example, the partial pressure of oxygen in Earth’s atmosphere at sea level is about 21% of the total pressure. This indicates that 21% of the molecules are oxygen.
Gas | Symbol | Content | ||
---|---|---|---|---|
Nitrogen | N2 | 78.084% | ||
Oxygen | O2 | 20.947% | ||
Argon | Ar | 0.934% | ||
Carbon dioxide | CO2 | 0.035% | ||
Neon | Ne | 18.182 parts per million | ||
Helium | He | 5.24 parts per million | ||
Methane | CH4 | 1.70 parts per million | ||
Krypton | Kr | 1.14 parts per million | ||
Hydrogen | H2 | 0.53 parts per million | ||
Nitrous oxide | N2O | 0.31 parts per million | ||
Carbon monoxide | CO | 0.10 parts per million | ||
Xenon | Xe | 0.09 parts per million | ||
Ozone | O3 | 0.07 parts per million | ||
Nitrogen dioxide | NO2 | 0.02 parts per million | ||
Iodine | I2 | 0.01 parts per million | ||
Ammonia | NH3 | trace |
Nitrogen
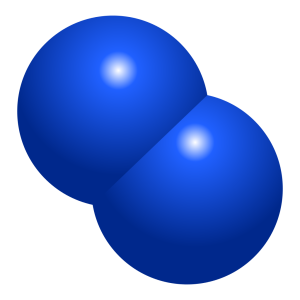
Nitrogen is the most abundant gas in Earth’s Atmosphere. It occurs as N2 molecules that are relatively unreactive. Most of the time we are unaware of this largest component of our Atmosphere. However, nitrogen is a critical part of all living things, and therefore the Biosphere must capture nitrogen from the Atmosphere. This can occur in two ways. Nitrogen can be oxidized by lightning in the Atmosphere, which combines it with oxygen to make nitrate (NO3–) ions. Alternatively, it can be reduced, by biological processes that occur, for example, in the roots of certain plants, to make ammonium (NH4–) ions. Both processes make nitrogen more accessible to living organisms. These processes are important parts of the nitrogen cycle, on which all life depends.
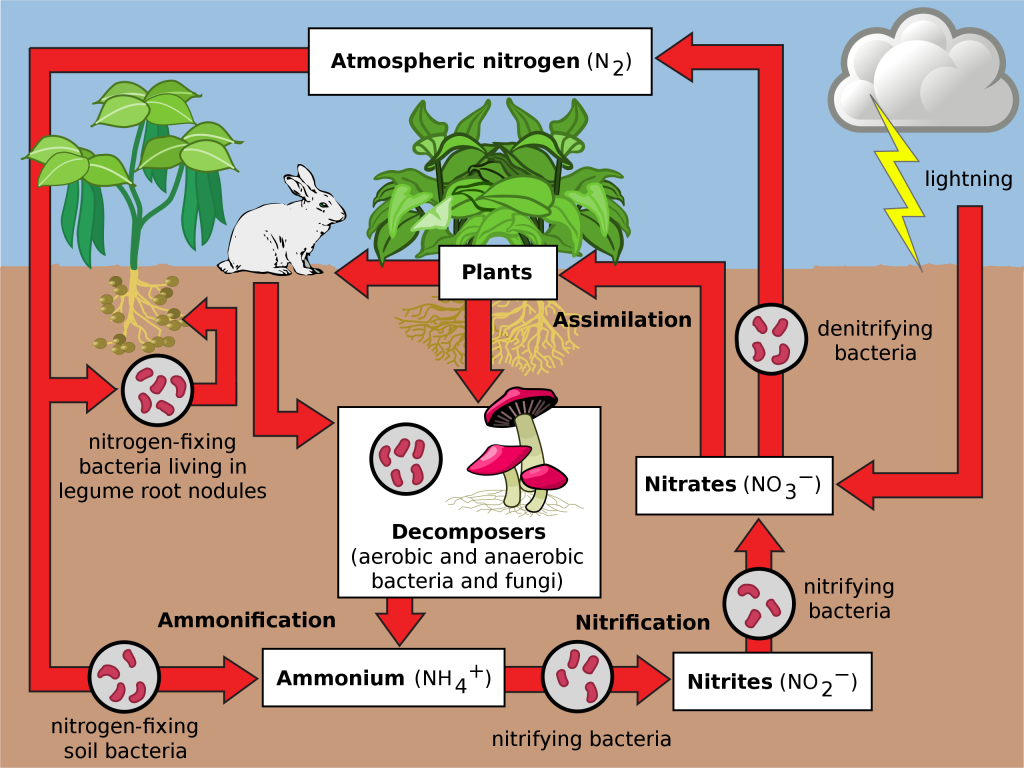
Oxygen
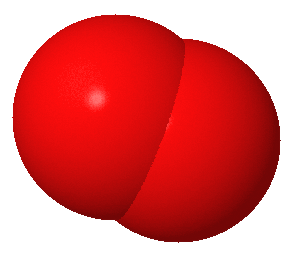
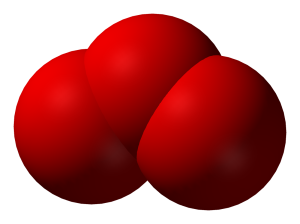
Oxygen represents about 21% of Earth’s Atmosphere at the present day, and is the second most abundant component. Oxygen is essential to all animal life and many plants, which obtain their energy by reacting oxygen with carbon compounds, a process known as respiration. The element oxygen actually occurs as two different gases in the Atmosphere.
Most oxygen exists as molecular oxygen (O2), consisting of two oxygen atoms joined by a double bond, but a small proportion exists as ozone (O3) in which three oxygen atoms are joined. Ozone forms by the action of ultraviolet radiation on oxygen in the Atmosphere, but the ozone that forms is even more effective at absorbing ultraviolet radiation, particularly at the shorter wavelengths that are most harmful to human and other animal life at the Earth’s surface. Ozone is most abundant in the stratosphere between 15 and 50 km above the Earth’s surface, though at maximum it typically only accounts for about 10 parts per million in the Atmosphere.
Water vapour
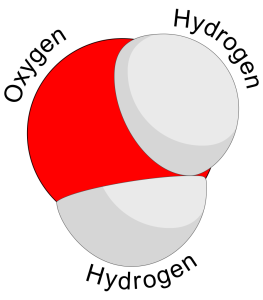
Water vapour[1] is the most variable component of Earth’s Atmosphere, but in most parts of the Atmosphere it’s the third most abundant gas, after oxygen and nitrogen. The average partial pressure of water is 2 to 3% of atmospheric pressure. However, very warm air may hold over 4% of water vapour, while in very cold air, the partial pressure of water vapour may be as low as 0.01%. Because water vapour pressure is so variable, it’s common to express the composition of the rest of the Atmosphere as “dry” percentages, after water has been removed.
For any given temperature, there is a saturation vapour pressure that represents the maximum amount of water vapour that can exist in the air if it’s in equilibrium with liquid water or (below freezing) with solid ice. Under most circumstances, if the partial pressure of water rises much above this saturation point, liquid water or solid ice will start to condense.
Water vapour is an important greenhouse gas, but because its saturation behaviour leads to frequent return of water from the Atmosphere to the Hydrosphere by precipitation, it is a less serious concern than carbon dioxide (below) and methane.
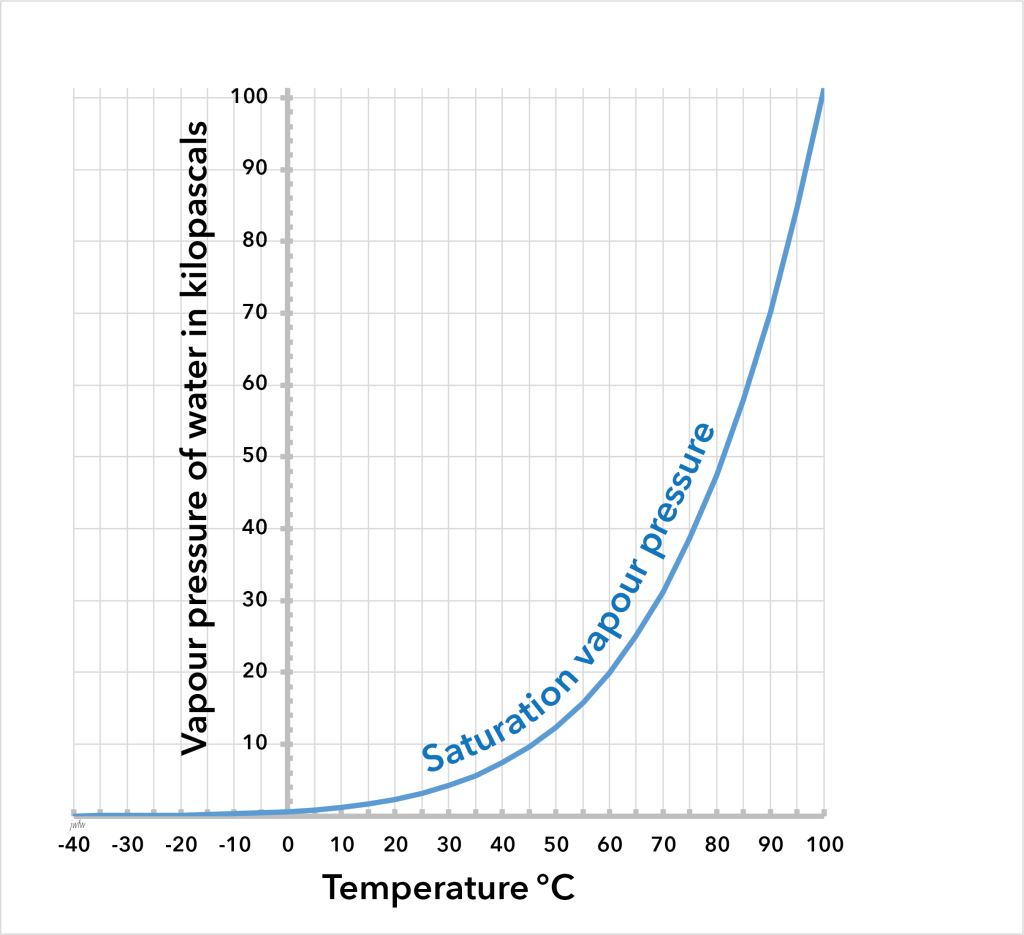
Argon
The next most abundant component, argon, is an inert gas: – one with eight electrons in the outer shell of its atoms, with the result that it participates in almost no chemical reactions. Argon accounts for about 1% of the gas in the Atmosphere, but because of its inert character, it does not participate in significant interactions with the other spheres.
Carbon dioxide
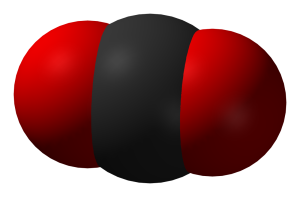
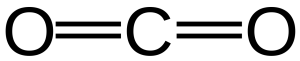
After argon, the next most abundant component is carbon dioxide or CO2, an important greenhouse gas. The abundance of carbon dioxide over most of the past 10,000 years (based mainly on measurements of air bubbles in glacier ice of known age) was about 280 parts per million, or 0.028 %. However, in the last 200 years, the amount of CO2 has been rising steadily to a present-day (2023) value of nearly 420 ppm.
Carbon dioxide has the ability to dissolve in water, producing an acidic compound called carbonic acid by the reaction:
CO2 + H2O = H2CO3
Carbonic acid undergoes further reactions once it’s dissolved in water, which we look at in more detail in the chapters on the hydrosphere.
Daily, weekly and monthly measurements of atmospheric CO2 are available from an observatory at Mauna Loa in Hawaii.
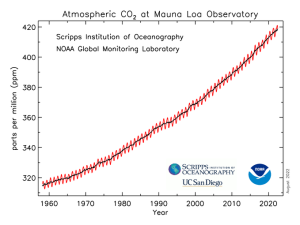
Other gases
Three of the next four gases by abundance are inert gases, which play passive roles in the Atmosphere similar to that of Argon. They are
- Neon 18 ppm (parts per million)
- Helium 5 ppm
- Krypton 1.1 ppm
Just ahead of Krypton in abundance is methane CH4 at 1.8 ppm. Methane is produced by natural processes in the Biosphere, but atmospheric methane is also increasingly contributed by the accidental release of natural gas, of which it is the main constituent. Methane is a potent greenhouse gas, second in importance to carbon dioxide. Its contribution to warming of the Atmosphere is less than that of carbon dioxide because its absolute contribution is low, and because it undergoes slow natural oxidation by reacting with oxygen, giving it a lower residence time.
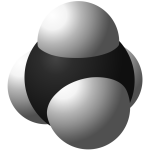
There are of course many other gases present in the Atmosphere in trace amounts, some of which we will meet in other sections as we look at human impacts on the Earth system.
Aerosols
In addition to gases, the Atmosphere contains very small particles of solids and liquids. If they are small enough, these particles may remain suspended in the Atmosphere for long periods of time, as they are continually buffeted by the fast-moving gas molecules. These small solid particles and liquid drops are known as aerosols. They include:
- Water droplets
- Ice particles
- Solid particles of rock and other dust
- Small particles of carbon derived from forest fires
- Particles of salt derived from the oceans
- Etc.
Water in the Atmosphere
Phases of water
Water is an unusual substance. It can exist at the Earth’s surface as three different phases. (Phases are materials that are separated from each other by distinct boundaries.) The three phases of water are water vapour, liquid water, and solid ice. In the Atmosphere, water vapour is part of the gas phase. Liquid water and ice exist in the Atmosphere as separate phases as aerosols, small particles that are suspended within the air.
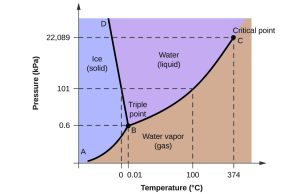
As pressure and temperature change in the Atmosphere, phase changes occur. Ice melts to produce liquid water. Water evaporates to produce water vapour. Ice may sublimate to produce water vapour. Each of these phase changes requires input of energy to break the bonds between the water molecules. This energy is called latent energy, or latent heat, meaning energy that is added or removed without changing the temperature. For example, it takes about the same amount of energy to warm ice from -16 °C to zero degrees Celsius as it does to bring ice at 0°C to water at 0°C.
Changes in the opposite direction release latent energy. Water freezes to produce solid ice. Water vapour condenses to produce either liquid water or solid ice. Each of these phase changes requires the removal of latent energy as bonds between water molecules are made. In thermodynamics, latent energy is explained as the energy that must be supplied to go from a more ordered, or lower entropy state (like ice), to a more disordered or higher-entropy state like water vapour.
Equilibrium between phases
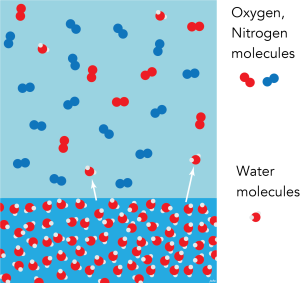
The figure shows a body of liquid water (maybe a lake) in contact with a gas (maybe the Atmosphere). The two have the same temperature, so the average kinetic energy of the molecules is the same, but the energy of individual molecules varies a great deal. Every so often, a molecule of water has enough energy to free itself from the surface of the liquid and enter the gas as water vapour. The partial pressure of water vapour in the gas will gradually increase.
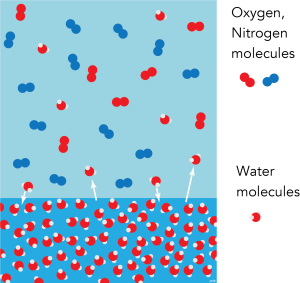
However, the molecules in the gas also have a range of kinetic energies, and sometimes the energy of a molecule of water vapour will be sufficiently low that, when it hits the surface of the liquid water, it will return to the liquid phase. A state of equilibrium will be reached when the rate transfer of molecules is the same in each direction. We say that the air is saturated with water vapour. If the water-vapour content of the Atmosphere increases beyond this level, it will be counteracted by increased condensation (provided there is a water surface available).
Saturation vapour pressure
The partial pressure of water vapour that can exist in equilibrium with liquid water varies with temperature, and is measured by the saturation vapour pressure. At 20°C the saturation vapour pressure of water is about 2.3 kPa or 0.023 atm, meaning that water makes up a little more than 2% of the molecules in saturated air at this temperature. At 40°C this value rises to 7.3%. At freezing point it is only 0.6%. Below freezing, the saturation vapour pressure is usually expressed relative to ice; it continues to fall with falling temperature. By minus 20°C, air is saturated when it contains only 0.1% of water vapour molecules.
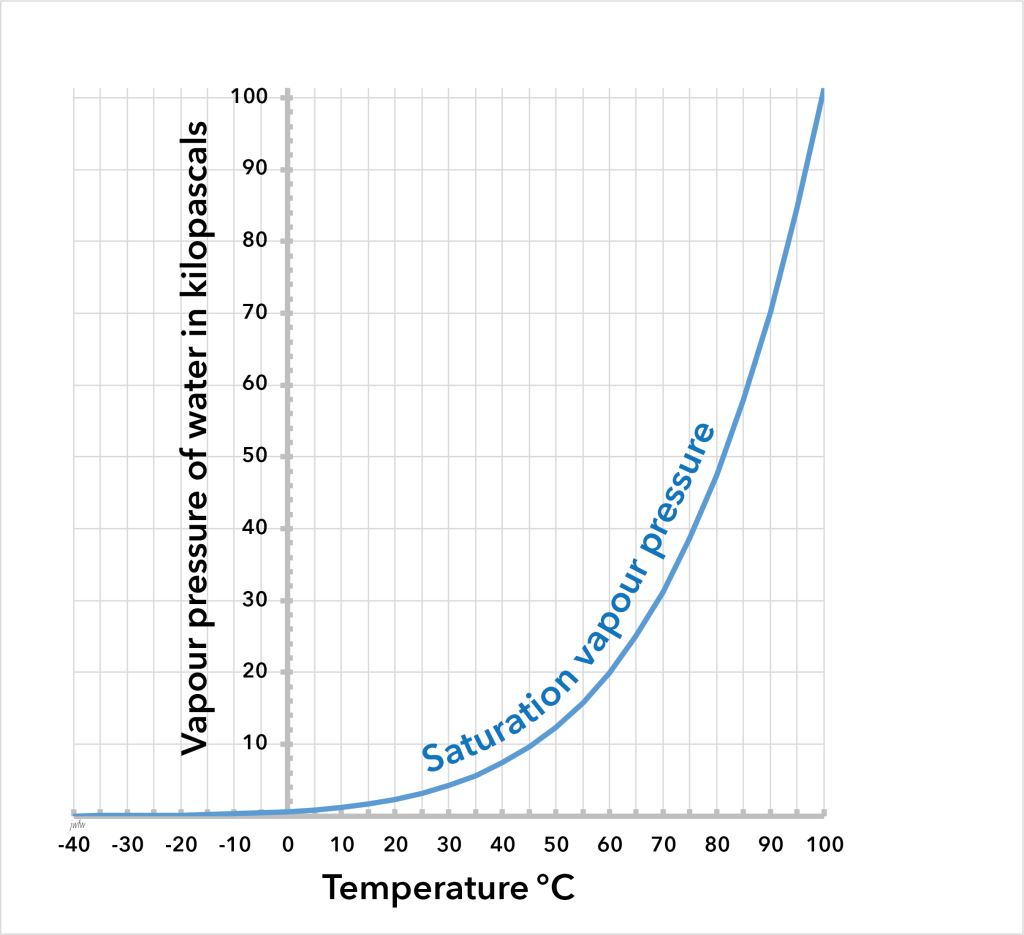
Relative humidity
Often, air in the Atmosphere is not saturated with water vapour. For example, in continental interiors far from the sea the air is often quite dry. If the water vapour pressure is only half as large as the saturation vapour pressure, we say that the relative humidity is 0.5 or 50%.
So
Relative humidity = (Water vapour pressure) / (Saturation vapour pressure)
Dew point
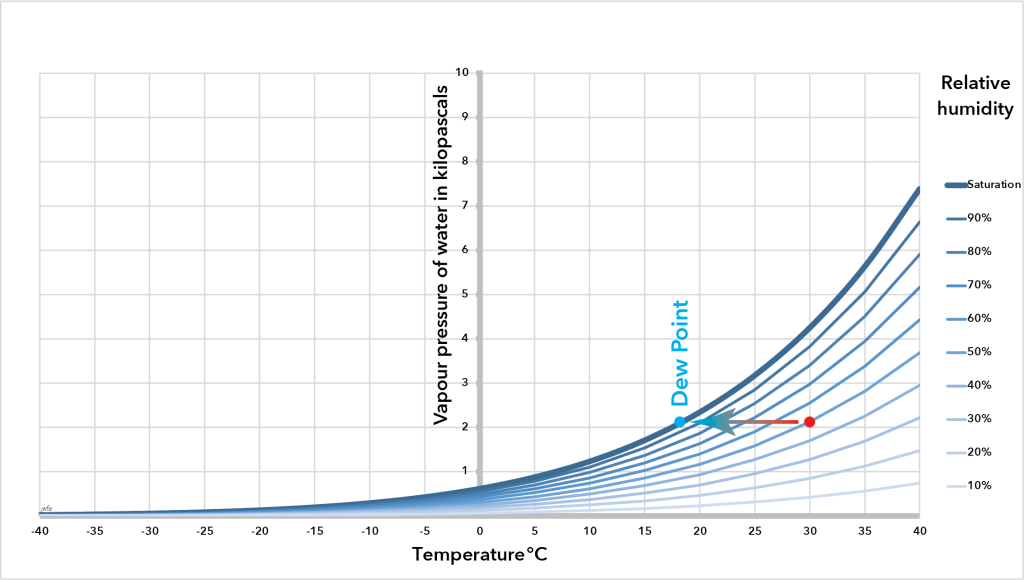
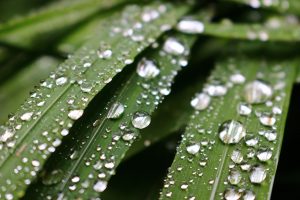
As temperature falls the saturation vapour pressure of air also falls. Therefore, if a body of unsaturated air is cooled, it eventually gets to a temperature where the relative humidity hits 100% – the air has become saturated as a result of falling temperature. We say the air has reached its dew point – the temperature that is cold enough for a deposit of water, known as dew, to form on exposed surfaces.
Condensation of water and ice
What happens when there is no surface available for condensation, for example high in the Atmosphere? The formation of a first, very small droplet of water is difficult, because the molecules in small drops with curved surfaces are more loosely bound than those in a large body of water, so their vapour pressure is a little higher than the saturation pressure. As the temperature falls, therefore, the air may become oversaturated. Extra energy must be taken out to achieve the first condensation of a very small water drop. That energy is known as the nucleation energy. As a result, condensation may not start until the temperature has fallen below the dew point.
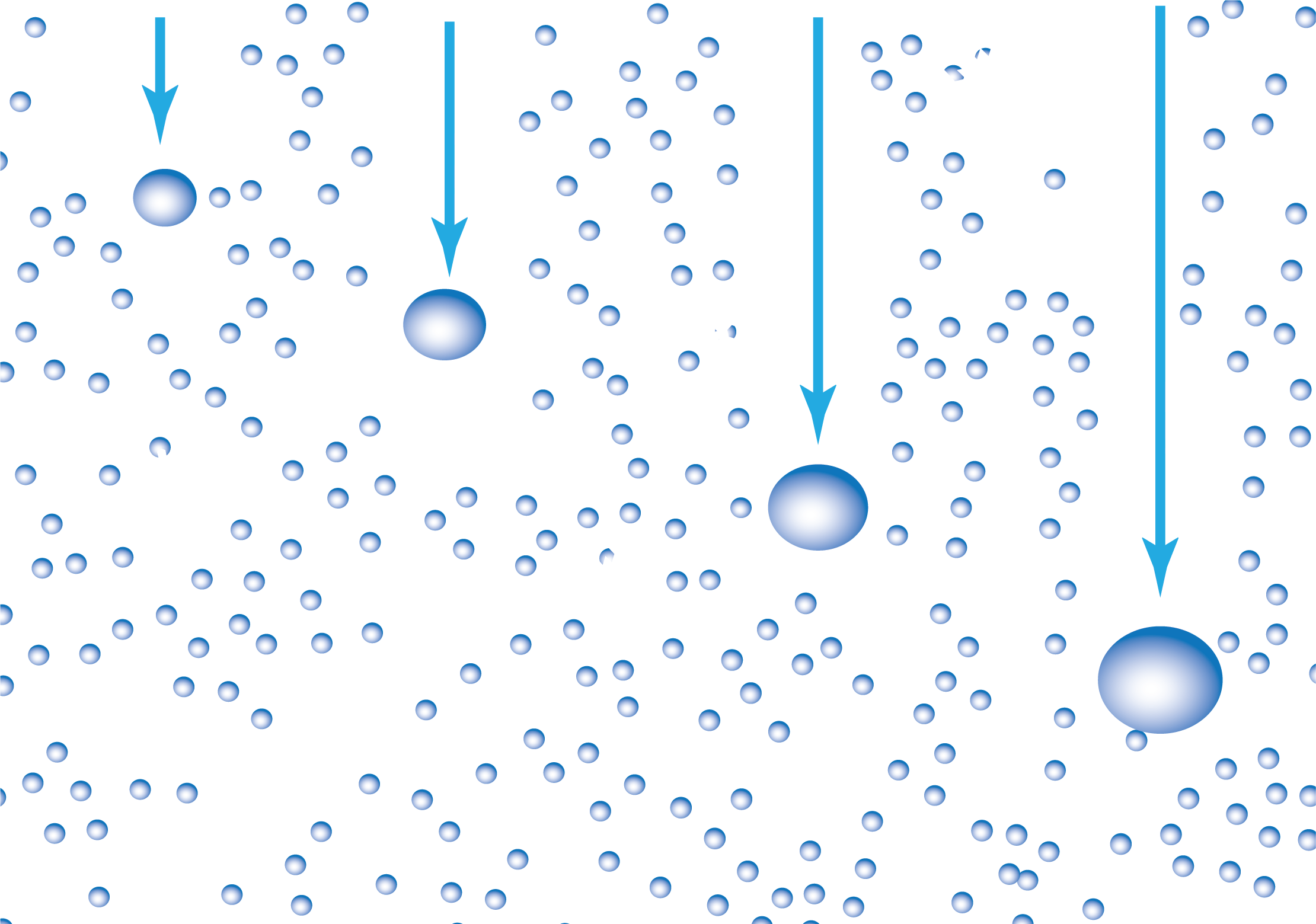
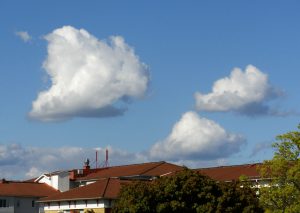
Once nucleation starts, water droplets grow rapidly to about 20 μm in diameter. The aerosol typically scatters the light, forming clouds. If there’s more than one drop per cubic millimetre of Atmosphere, frequent collisions between droplets form larger drops that are no longer suspended, and those raindrops fall as rain.
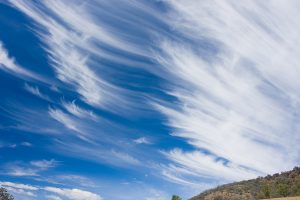
If the temperature is below freezing. The nucleation of ice crystals is particularly difficult. Although the temperature may fall well below freezing, the high nucleation energy of ice inhibits ice formation. So supercooled water droplets form instead, producing freezing mist (which may deposit ice on any surface that it touches). Once the temperature becomes low enough for the nucleation of ice, the supercooled water droplets release vapour which then condenses on the ice crystals, allowing them to grow. This is known as the Bergeron process. If the ice crystals become large enough that they are no longer suspended in the Atmosphere, then snow will fall.
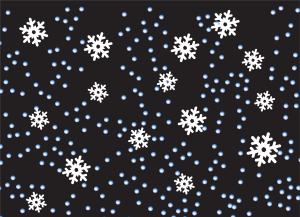
Adiabatic lapse rate of condensing air
In an earlier section. We saw that as warm air rises, it tends to cool adiabatically by about 10°C/km. However, if condensation starts, it releases latent energy as heat which offsets the adiabatically cooling. For air that is actively condensing the rate of cooling is reduced to about 6°C/km. This is known as the moist adiabatic lapse rate.
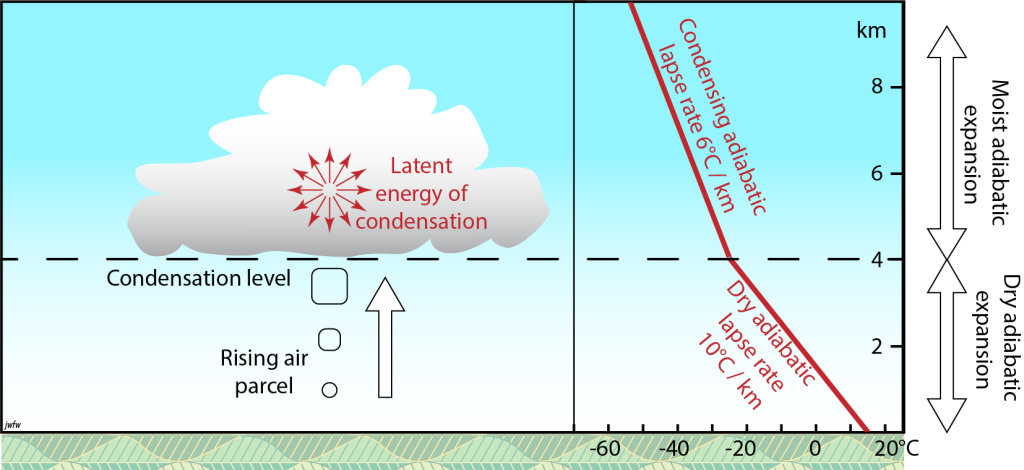
- Spelled 'vapor' in the USA ↵
The envelope of gas surrounding a planet
The third planet from the Sun known for its similar size to Earth; the brightest planet in Earth's night sky
Relating to planet Jupiter and the other outer planets.
The fourth planet from the the Sun
The nearest planet to the Sun, and the smallest planet.
Short-term changes in Earth’s atmosphere
The lowest part of Earth’s atmosphere directly in contact with the surface of the Earth, which warms it from below, leading to an upward decrease in temperature. The troposphere contacts the stratosphere at the tropopause.
Atmospheric scientists who study short term changes in the atmosphere known as weather.
Long-term trends in Earth’s atmosphere which may affect weather, but take effect for much longer. Climate is weather averaged over periods longer than a single year
An atmospheric scientist who studies long-term changes in the Atmosphere
Element 7. In the molecular form N2, the most abundant gas in Earth’s atmosphere.
Element 8. In the molecular form O2, the second most abundant gas in Earth’s atmosphere.
A measure of the average kinetic energy of the atoms or molecules in a material, typically measured on the Celsius (°C) or Kelvin (K) scale
The lowest possible temperature, at which molecules have no kinetic energy
The total thermal kinetic energy of all the molecules in a body or system
A value which is independent of the amount of material under consideration, such as temperature or chemical concentration.
A quantity, such as heat energy or mass, which varies in proportion to the amount of material considered
The force per unit area exerted by a fluid on its surroundings
Mass per unit of volume of a substance.
A change to a system is adiabatic no heat energy is gained or lost during the change
The rate at which air cools with elevation as it rises adiabatically (without heat transfer) within the atmosphere. The adiabatic lapse rate may be expressed in degrees per kilometre of elevation.
The layer of the Atmosphere above the Mesopause, where temperature increases with elevation.
The boundary separating the mesosphere and thermosphere, where the temperature of the atmosphere reaches its lowest point
The layer of Earth’s atmosphere directly above the stratosphere, divided from it by the stratopause. In the mesosphere, the temperature decreases with elevation.
An atom or group of atoms that has lost or gained one or more electrons, resulting in a net positive or negative electric charge
The layer of Earth’s atmosphere, including the mesosphere and thermosphere, where solar radiation may add or remove electrons from atoms, creating ions
Visible light produced in the northern hemisphere by solar wind’s interaction with particles in Earth’s thermosphere; northern lights
Visible light produced in the southern hemisphere by solar wind’s interaction with particles in Earth’s thermosphere; southern lights
A compound consisting of three oxygen atoms O3 which exists in small amounts in the atmosphere due to both natural and anthropogenic activity.
Electromagnetic radiation with wavelength slightly shorter than visible light, and frequency slightly higher, lying beyond violet in the electromagnetic spectrum.
The boundary dividing the mesosphere and stratosphere in Earth’s atmosphere. Here, the temperature reaches its peak between the two layers.
A zone of upward-increasing temperature in the atmosphere, between the tropopause, below, and the stratopause, above.
The boundary lying between the troposphere and the stratosphere, where temperatures reach a minimum after decreasing with altitude in the troposphere, and before increasing once again in the stratosphere.
The pressure exerted by a single gas within a gas mixture; the partial pressure of each gas is proportional to its fraction of molecules in the mixture.
The combination of an element with oxygen, or more generally, any chemical process resulting in electron loss
The removal of oxygen or the addition of hydrogen to an element or compound, or, more generally, any chemical reaction that results in a gain of electrons
The oxidation of reduced (organic) carbon compounds by an organism to yield energy, carbon dioxide and water
Water in its gaseous state, a colourless invisible gas
The maximum concentration of water vapour in air when at equilibrium with liquid water or solid ice.
An inert gas, element 18
A gas in which each molecule combines one atom of carbon with two of oxygen; formula: CO2
A compounds in the Earth’s atmosphere which absorbs infra-red radiation, preventing or delaying its escape into space.
A weak acid formed in solution when carbon dioxide dissolves in water: formula H2CO3;.
A gaseous compound of carbon and hydrogen CH4. Methane is the principal component of natural gas. In the Earth's atmosphere it acts as a greenhouse gas.
The average time that a molecule remains in a reservoir; obtained by dividing the size of the reservoir by the flux in or out of the reservoir
Small particles or droplets suspended in the atmosphere.
The part of a system that is separated from other parts by distinct boundary surfaces; for example water exists in three phases: solid ice, liquid, and vapour, which do not mix.
The phase change of solid directly to gas, without liquid as an intermediary step.
Energy released or absorbed in phase changes.
The amount of energy in a system that is not available to do physical work; also a measure of the degree of disorder in a system.
The vapour pressure of water in air at a given temperature divided by the saturation vapour pressure at the same temperature.
For an undersaturated body of air, the dew point is the temperature at which the air would become saturated. The dew point is always equal to or lower than the actual temperature of the air.
Describes a situation where a substance (e.g. water vapour) is more concentrated in a mixture (e.g. air) than the amount that would be in equilibrium with a separate phase (e.g. liquid water or solid ice)
Energy required to form the first stable particle or droplet of a substance (usually water or ice fomed during a condensation process)
A visible mass of aerosol water droplets suspended in the Atmosphere
A typical process for the formation of snow. Vapour condenses first as supercooled water droplets. As the temperature falls the droplets release vapour which condenses as ice.
Ice crystals formed by condensation directly from water vapour, that fall as precipitation
The rate of cooling as mass of moist air rises without exchanging heat with its surroundings, while water vapour in the air condenses. The rate of adiabatic cooling is offset by the release of latent energy during condensation