15 Structure and Growth of Living Organisms
This chapter is a pre-review version
Learning Outcomes
By the end of this chapter you should be able to:
- Distinguish prokaryotic and eukaryotic cells;
- Describe the roles of main categories of biological compounds;
- Distinguish nutrients and toxins;
- Describe the cycling of key elements through the Biosphere;
- Identify processes of biomineralization.
In this section we look at the growth and structure of living things, focussing on the basic materials that living things use. As we shall see in the next chapter, many of these characteristics must have developed very early in the evolution of life on Earth.
Cells
Eukaryotes and prokaryotes
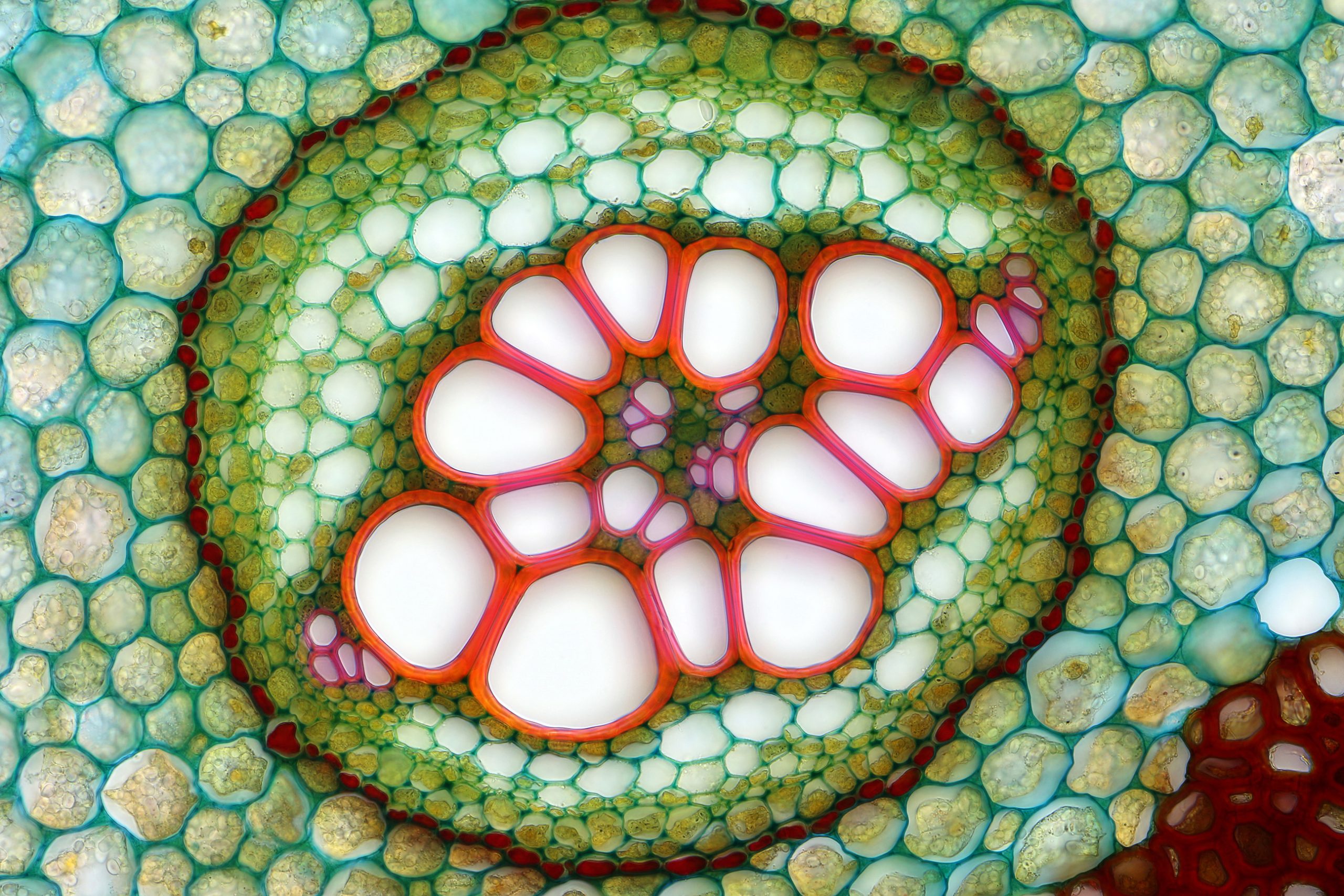
Most large organisms are divided into cells, and are therefore termed multicellular. The cells of most living organisms are typically 0.01 to 0.05 mm in diameter, but some single cells — notably the yolk cells of bird’s eggs — reach up to a few centimetres in diameter. The cells of multicellular animals and plants have several things in common: in each cell is a nucleus where nucleic acids such as DNA are concentrated. In the surrounding material, termed cytoplasm, there are organelles — structures with particular functions, like mitochondria, where many of the reactions of respiration take place, and chloroplasts in plants, which are responsible for photosynthesis.
However, many very small organisms consist of a single cell, and it is likely that life started off as such unicellular organisms.
When we look closely at unicellular organisms we find we can further subdivide them into two grades.
Some have a cell structure very similar to that of multicellular organisms, with a nucleus, cytoplasm, and organelles. Such cells are known as eukaryotic, and the organisms that they make up are called eukaryotes, regardless of whether they are unicellular or multicellular.
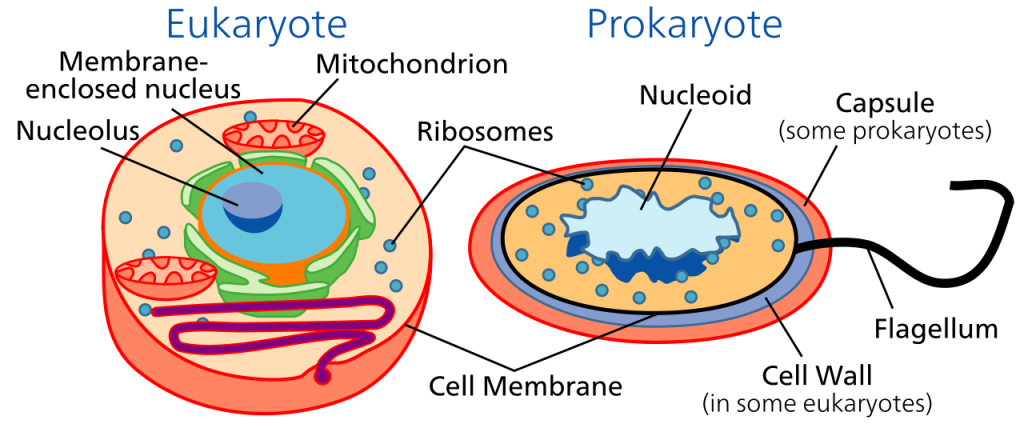
In contrast, some unicellular organisms lack the distinct nucleus of the eukaryotes, and also lack most of the organelles. Such organisms are called prokaryotes. All prokaryotes are unicellular.
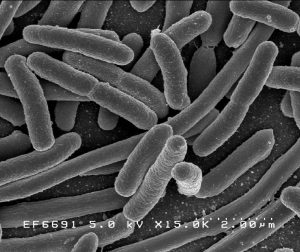
The Prokaryotes include two large groups.
- Bacteria (singular bacterium). Some of these cause diseases in multicellular organisms. Others, known as cyanobacteria (formerly blue-green algae) are are responsible for a lot of the photosynthesis that takes place on shallow tropical continental shelves where carbonate sediment is deposited.
- Archaea (singular archaeon) are bacteria-like unicellular organisms many of which use unusual energy sources such as methane, ammonia, or metal ions coming from hot springs on the sea floor. In images, Archaea and Bacteria appear very similar, and Archaea have only recently been distinguished as a separate group, characterized by some fundamental differences in body chemistry. For example, the cell membranes of Archaea contain a different type of phospholipid from Bacteria.
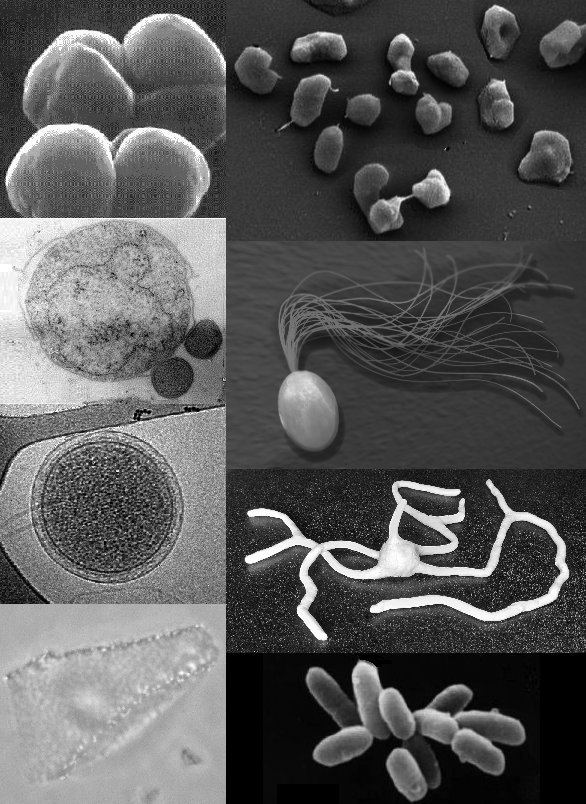
Eukaryotes have cells with a distinct nucleus, which contains most of the genetic material, DNA, surrounded by cytoplasm containing organelles that carry out specific functions within the cell. For example, mitochondria handle respiration processes, and chloroplasts carry out photsynthesis in eukaryotic plants. It’s likely that the first eukaryote evolved from a member of the Archaea which began to live in conjunction with bacteria in a cooperative relationship called symbiosis. Eventually the archaea engulfed the bacteria. The included bacteria became organelles like mitochondria and chloroplasts in the cytoplasm of the resulting eukaryote. Eukaryotes can be divided into two large groups:
- Unicellular eukaryotes are also known as protists.
- Multicellular eukaryotes include all the larger animals and plants that we, as humans, are most familiar with.
Reproduction of cells
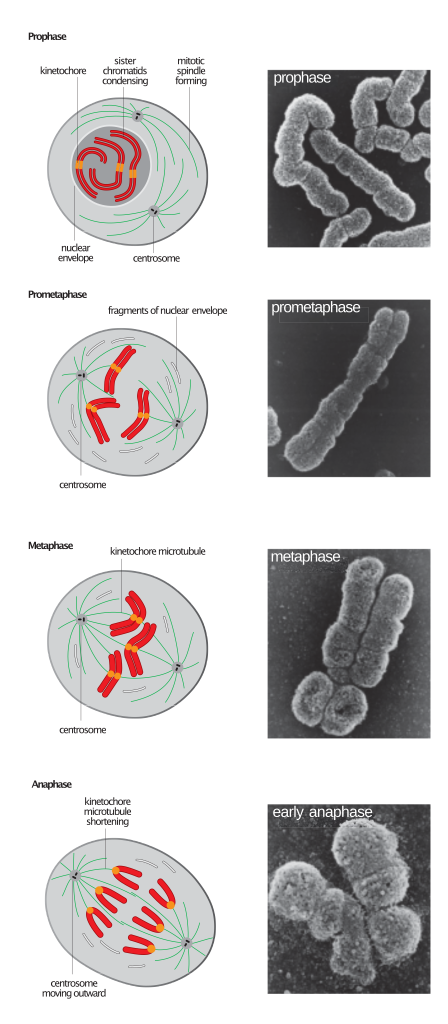
All unicellular organisms reproduce by cell division. In this process, the genetic material (carried in the nucleic acid DNA) is copied first, and then the resulting copies are distributed into two parts of the dividing cell. This type of cell division, known as mitosis, allows unicellular organisms to reproduce, but in multicellular organisms its main function is to allow the whole organism to grow.
Sexual reproduction involves the joining two different cells (known as fertilization) to produce a new cell with a double complement of DNA – a double number of genes. It takes place in both unicellular and multicellular organisms. One thing that has to happen whenever sexual reproduction takes place is that the double genes have to be reduced in number so that the amount of DNA doesn’t go on doubling in each generation. This is achieved by a special type of cell division called meiosis in which cells divide while halving the amount of genetic material. In most multicellular animals, meiosis takes place in the production of eggs and sperm, halving the amount of DNA before fertilization doubles it again. In most plants and unicellular organisms, fertilization takes place first and is followed by meiosis.
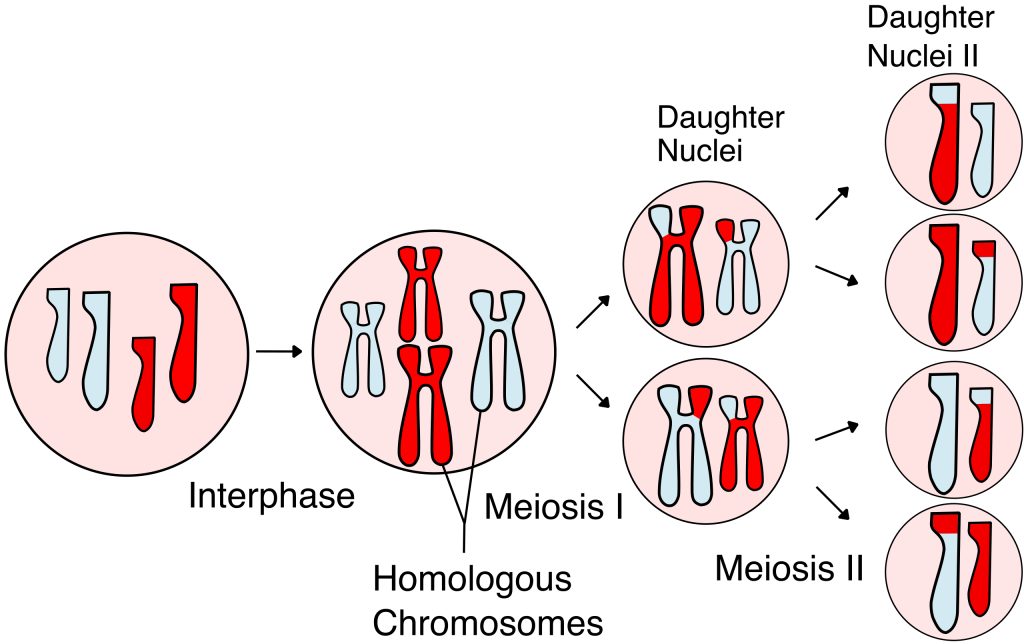
Biological compounds
We have mentioned some common biological polymers (chain molecules with repeating elements), such as protein and DNA, so we should look systematically at the chemical compounds that make up the majority of the cells of all organisms.
All known cells have some common types of molecules built from reduced carbon, oxygen, hydrogen, and other elements. These include
- Proteins
- Nucleic acids
- Lipids
- Carbohydrates
and many more.
Proteins
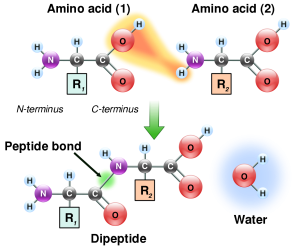
Proteins are polymers of carbon (C), hydrogen (H), nitrogen (N), oxygen (O) and other elements. They make much of the structure of cells and organelles, including the membranes that separate cells and organelles from their surroundings. They are built from units called amino acids, of which about 20 different types are common in living cells. Amino acids are linked together in chains by carbon–nitrogen peptide bonds, so nitrogen is an essential component of life. The exact sequence of amino acids causes protein molecules to twist into particular three-dimensional shapes, which carry out particular functions within cells. Certain amino acids contain sulfur; these are particularly important in controlling the shape of a protein molecule, because they allow the chain to bond to iteslf through disulfide bonds.
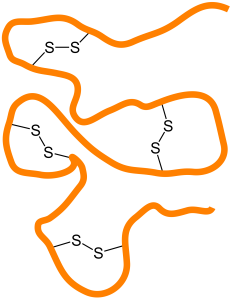
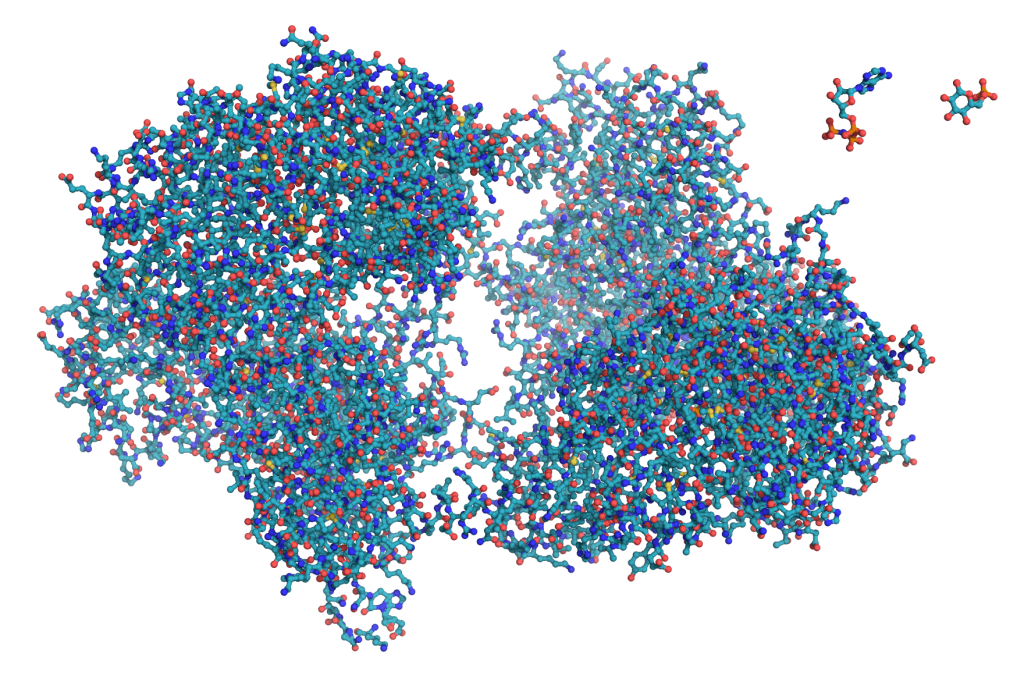
Nucleic acids
Nucleic acids, as their name implies, are concentrated in the nuclei of eukaryotic cells, but they are found in prokaryotes too. Two types of nucleic acid are important in the building of proteins and in reproduction of cells.
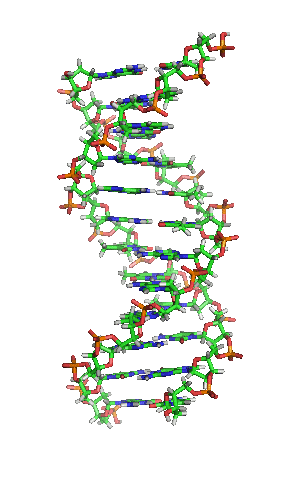
Deoxyribonucleic acid or DNA
Deoxyribonucleic acid or DNA consists of two chains involving carbon, oxygen, hydrogen, nitrogen, and phosphorus (C, O, H, N and P) that spiral around each other. Molecules called bases form bridges between the two chains, making a spiral ladder structure known as a double helix. Each “rung” of the ladder is made of two bases, and the bases are always paired in the same combinations, so that if the two strands of the double helix are separated, each one can form the basis for a new double helix. This is how the DNA gets replicated into both cells during mitosis.
DNA replication during mitosis. By I, Madprime, CC BY-SA 3.0, https://commons.wikimedia.org/w/index.php?curid=11427353
The sequence of bases in DNA carries a code for building a protein. Each group of three bases codes a different amino acid. How is this accomplished in a cell? This is where RNA comes in.
Ribonucleic acid or RNA
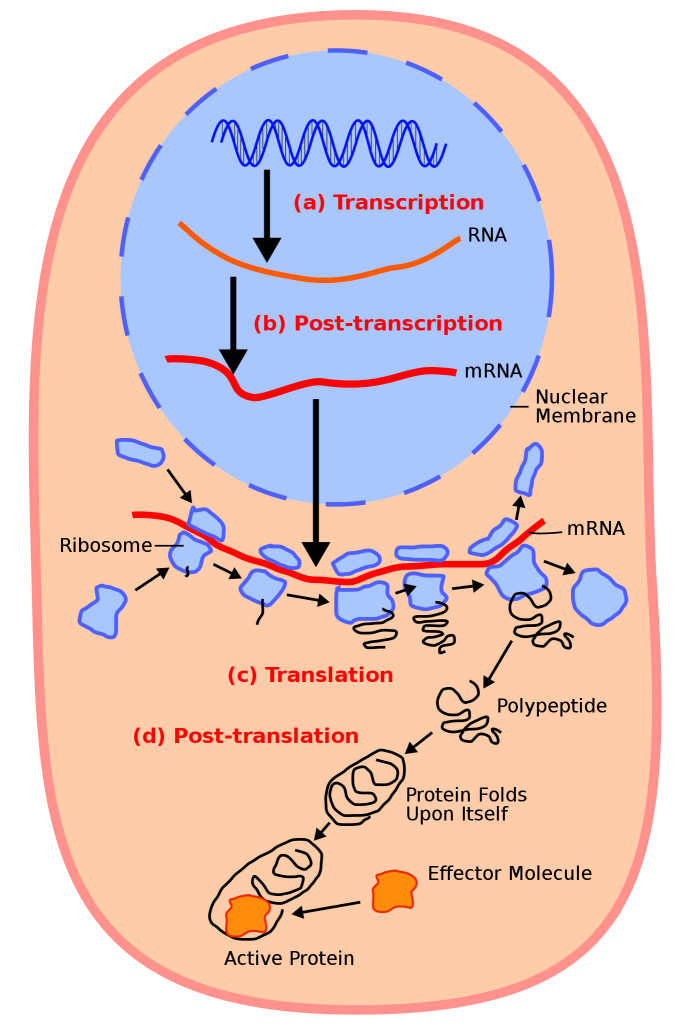
Ribonucleic acid or RNA has somewhat similar structure to DNA but does not readily form a double helix. However, when the strands of DNA become separated, a strand of “messenger RNA” can form on a DNA template, and the sequence of bases is mirrored onto the RNA strand. Once formed, the messenger RNA moves out of the nucleus (if the cell is eukaryotic) where it encounters small organelles called ribosomes. These are effectively molecular machines for building proteins based on the sequence of bases carried by the RNA.
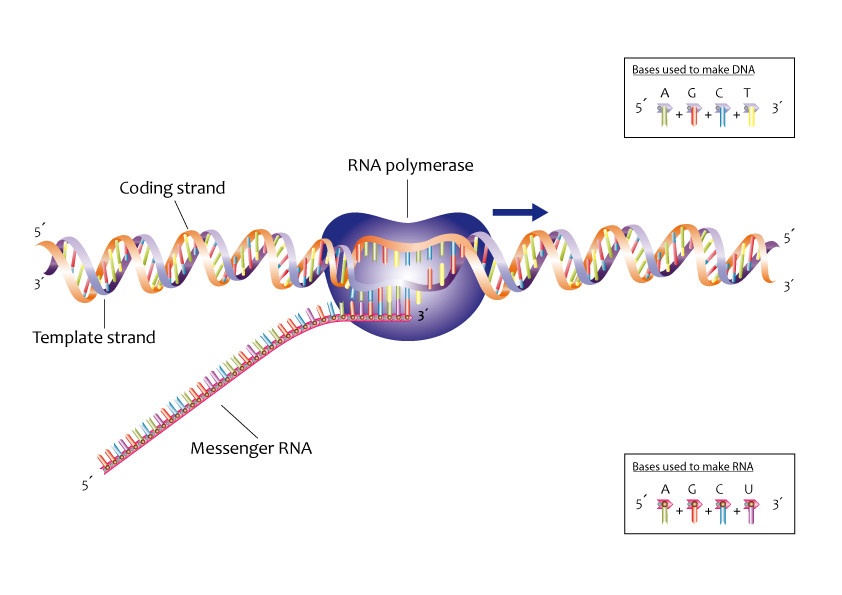
Lipids
In contrast to the components of proteins and nucleic acids, which are polar molecules and can therefore dissolve in water, lipids are non-polar molecules that do not dissolve in polar solvents like water. Lipids include waxes, fats and oils; the non-polar nature of these materials explains why they form separate droplets in water, rather than dissolving.
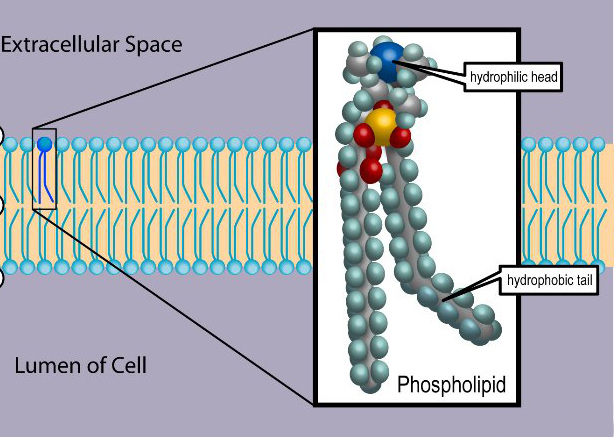
More complex lipids molecules that involve the element phosphorus (P), called phospholipids, have one end that repels water (hydrophobic) but the other end of the molecule is polar, and attracts water (hydrophilic). Phospholipids combine with proteins to make membranes – the thin layers of organic molecules that enclose cells and organelles. The combination of polar and non-polar materials helps to control the flow of other substances in and out of cells.
Structure of a cell membrane composed of a double layer of phospholipids. © 1999-2023, Rice University. Licensed under a Creative Commons Attribution 4.0 International License.
One of the ways that Archaea were first recognized as a distinct group from bacteria is that their phospholipid molecules have a branched structure that is distinctly different from that of other organisms.
Carbohydrates
Carbohydrates are relatively simple compounds of carbon, hydrogen, and oxygen. We already met simple carbohydrates such as the sugar, glucose. Some carbohydrates are polymers. Two are of particular importance in the biosphere:
- Starch is used by plants to store energy, and is therefore a major food source for heterotrophs, including humans/
- Cellulose is used by plants to build cell wals, and by bacteria to build thin membrane-like coatings called biofilms. Cellulose is the single most abundant organic substance in the biosphere.
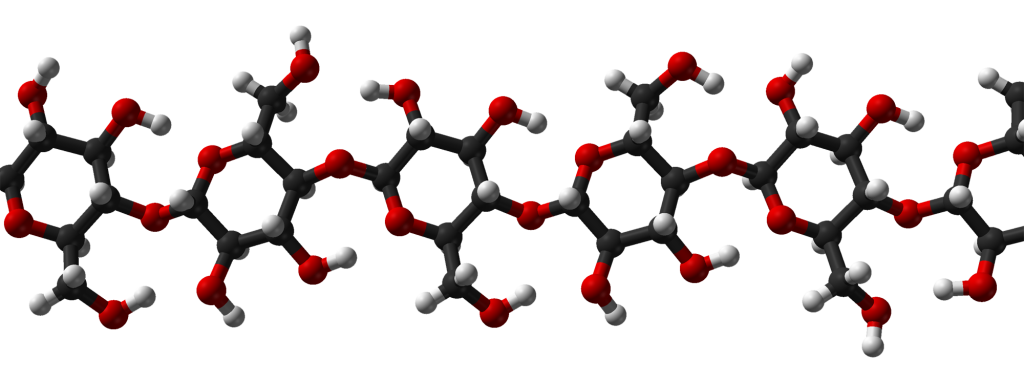
Nutrients, toxins, and cycles
Nutrients and toxins
From the previous sections, it is very clear that the vital elements of protein and DNA are essential to life. So far, we have mentioned carbon, oxygen, hydrogen, nitrogen, phosphorus, and sulfur (C, H, O, N, P, S), known as the “bix six” nutrients essential to all known living things. However, there are many other elements that are essential to living things, and which therefore can be considered nutrients. Some elements are required for just a few essential biological polymers, so although they are essential to life, they are required in tiny quantities. Such elements are known as micronutrients.
Because both the Hydrosphere and the outer parts of the Geosphere are variable in composition, there are environments where one or other of the micronutrients are in short supply. The growth of living things is then limited by one micronutrient. If the supply of that one nutrient changes, large changes in the populations of animals and plants can occur. Such nutrients are described as limiting nutrients
There are also elements that tend to break up biological polymers or interfere with their functions, and are therefore toxic to organisms. Such elements are sometimes described as toxins. However, the distinction between nutrients and toxins is not clear-cut: there are many elements which are essential to life in small concentrations but become toxic in higher concentrations. Also, the oxidation state (number of electrons added or removed) may affect the toxicity of certain elements. The periodic table below is shaded according to which elements are nutrients and which are toxins. Elements that can behave as both nutrients and toxins combine both types of shading.
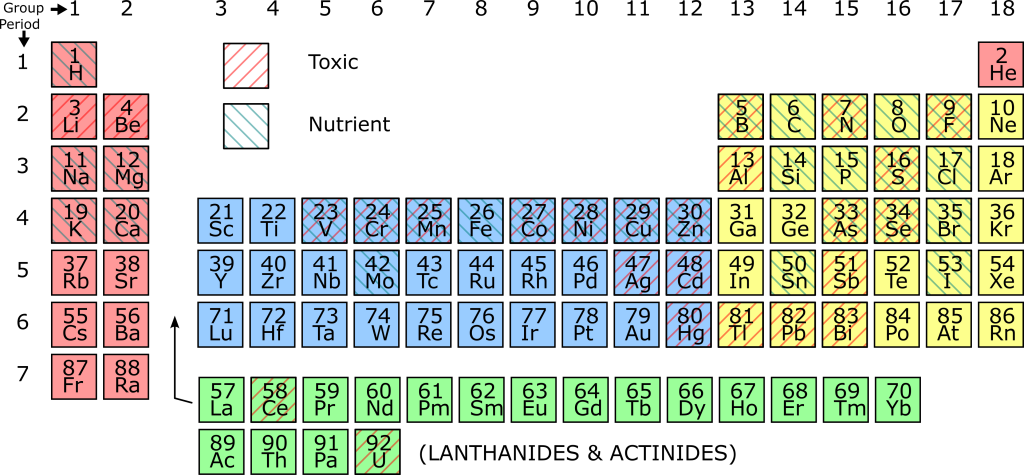
Each of the above biologically significant elements is involved in its own biogeochemical cycle, as it passes through different spheres of the Earth system. We have looked at the carbon cycle already. The cycles of nitrogen and phosphorus are particularly important.
Nitrogen cycle
Nitrogen (N) occurs as the largest component (78%) of Atmosphere as N2 molecules. The two nitrogen atoms are held together by a triple bond N≡N which is particularly difficult to break: the bond energy is 945 kJ/mol, greater even than the strength of the bonds that hold carbon dioxide together.
However, these triple bonds are not found in any of the many biological polymers that contain nitrogen. Instead, the nitrogen in the biosphere is derived from nitrogen that has either been oxidized or reduced from its atmospheric form.
- Oxidized nitrogen occurs linked to oxygen as nitrate ions NO3–
- Reduced nitrogen occurs linked to hydrogen as ammonium ions NH4+
Most of the nitrogen in important biological polymers is linked to hydrogen and is therefore part of the reduced category.
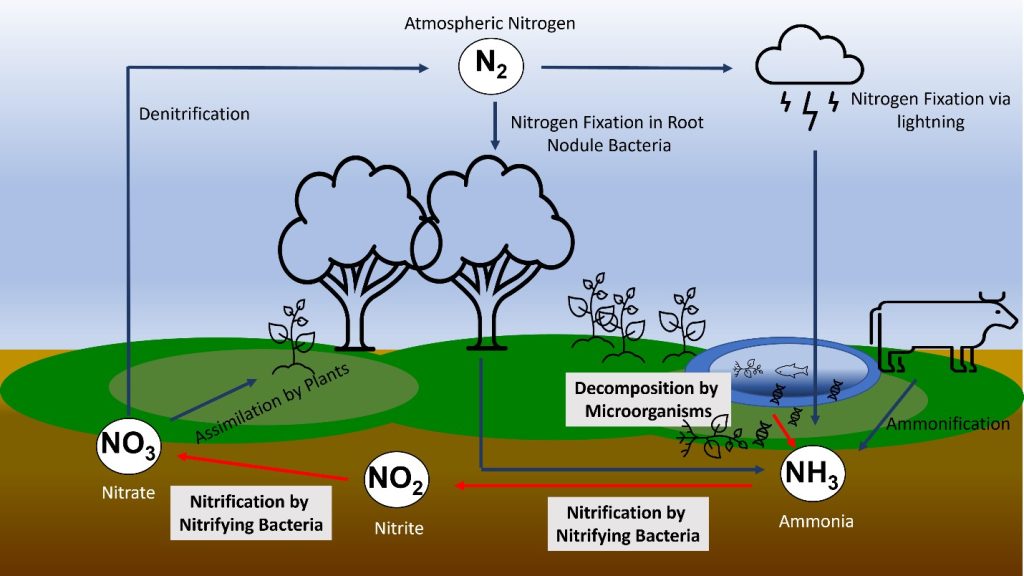
Nitrogen fixation
To make nitrogen available to the biosphere, it must be convertined to a reactive form a process known as nitrogen fixation. Two main natural processes operate in the modern Earth:
- Lightning provides enough energy to break up nitrogen molecules, allowing them to react with oxygen, eventually producing oxidized nitrate ions (NO3–). This process is thought to be responsible for fixing up to about 2 MT of nitrogen annually. Lightning was probably the main process of nitrogen fixation in the early Earth, although before the rise of atmospheric oxygen at about 2 Ga it would have operated differently, and may have delivered reduced nitrogen (in the form of carbon-nitrogen compounds) directly to the biosphere.
-
Root nodules on a soybean plant. By Isarnan – https://www.flickr.com/photos/91814899@N04/14691121542/in/photolist-aLgKi8-6rJT1G-pDscWi-5vWFhP-dV9ijm-pB4CUG-oocQB1-9hqZPF-Kk3jrd-dLNf1r-21d8jGJ-9hu8dw-9hqZVc-71nsEM-9hu8hG-9hvCVh-22eNCxi-59vwkN-EiSpDZ-dLTNw5-HRSgb5-hmPzbm-fJoPWg-6DKbQF-ANzCpC-2dsGx6X-x2oRD4-yjUSFy-82sjtg-ntG6uW-EFeTBH-228pakf-ANzF3d-nLbFkr, Public Domain, https://commons.wikimedia.org/w/index.php?curid=82770118 Certain groups of prokaryotes, belonging to both the bacteria and archaea have enzymes capable of reducing atmospheric nitrogen to produce ammonium ions and related organic molecules. However, this process takes a lot of energy. The most effective nitrogen-fixing microbes at the present day live in symbiotic relationships with plants, particularly legumes — principally peas and beans — where nitrogen-fixing bacteria live in nodules on the root systems. The nitrogen-fixing process must take place in the absence of oxygen, and the host plants provide a suitable anaerobic environment within the root nodules. (This suggests that nitrogen fixation probably evolved before the Earth’s atmosphere contained much oxygen). When the host plants die, much of the nitrogen ends up in the soil and the Hydrosphere, where it is accessible to other plants and animals. This process is responsible for delivering about 14 MT/yr of nitrogen to the biosphere.
The provision of nitrogen to crops has become extremely important to human agriculture, because nitrogen is a limiting nutrient for many crops:
- About an additional 10 MT of fixed nitrogen is produced industrially each year, primarily for the manufacture of agricultural fertilizers.
Humans and other heterotrophs acquire their nitrogen, together with their carbon, from autotrophs consumed as food in their food chain.
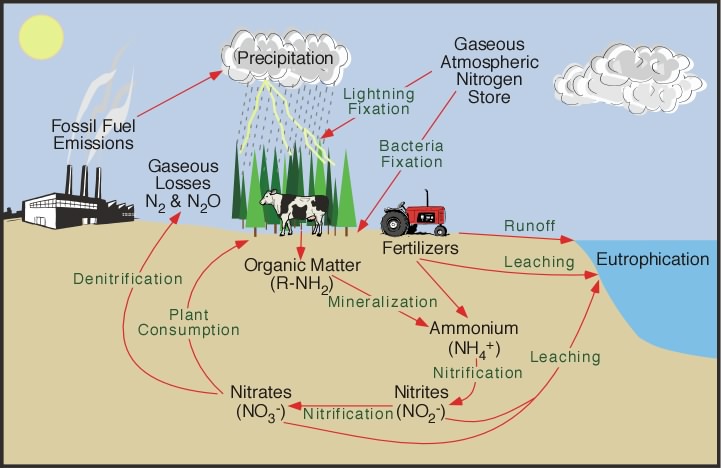
Denitrification
If nitrogen fixation continued without any return of molecular nitrogen (N2) to the atmosphere, the atmospheric nitrogen would be continuously depleted. However, the nitrogen fixation process is counterbalanced by denitrification carried out by aerobic bacteria, which react ammonium ions with oxygen to produce water and molecular nitrogen. These bacteria presumably evolved more recently than the nitrogen fixing microbes described above, as they depend on atmospheric oxygen.
As far as we know, denitrificationn eventually returns most of the naturally fixed nitrogen to the atmosphere. The impact of human industrial nitrogen fixation on atmospheric molecular nitrogen is quite small because the atmosphere is a very large reservoir of nitrogen (about 90% of the global amount). A small perturbation of the nitrogen cycle by humans has an imperceptible effect on the atmosphere. However, the impact of agricultural nitrate fertilizer on surface water may be dramatic. Any organisms for which nitrogen is a limiting nutrient are fertilized. In some cases the addition of agricultural nitrate fertilizer to rivers and lakes has produced a bloom of unicellular plants, contributing extra reduced carbon to the ecosystems, reducing the availability of oxygen. An additional concern resulting from human use of nitrate as fertilizer is that a proportion of the nitrogen is returned to the atmosphere not as molecular nitrogen but as nitrous oxide N2O, which is a potent greenhouse gas. Although nitrous oxide is produced naturally as a result of lightning, human fertilizer use has resulted in increases of atmospheric N2O, which add to the greenhouse effect of anthropogenic carbon dioxide and methane.
Biomineralization
Many organisms produce substances that remain after the organism has died. When these substances contribute significantly to sediment deposited on the sea-floor, lake floor, or land surface, we say that biomineralization has occurred. It’s possible to distinguish two types of biomineralization. Biologically induced biomineralization occurs when mineral material is deposited as a by-product of an organism’s life-processes. In contrast, biologically controlled biomineralization occurs when an organism has evolved to concentrate mineral material that serves in a particular role for the organism, such as a skeleton. Biomineralization produces several different types of mineral material.
Carbonate biomineralization
Calcium carbonate (CaCO3) is one of the most common products of biomineralization. Some of the earliest organisms on Earth, the prokaryotic Cyanobacteria, would become encrusted with calcium carbonate because the processes of photosynthesis create conditions of high pH that are conducive to the precipitation of calcium carbonate, and because calcium ions (Ca2+), abundant in sea water, become attached (“adsorbed”) to their outer cell sheaths. However, many of the most ancient sedimentary rocks contain abundant stromatolites — dome-and pillar-like layered structures of calcium carbonate built by Cyanobacteria, probably providing an evolutionary advantage by keeping the micro-organisms in clear water where they could access light. Thus the cyanobacteria probably advanced from induced biomineralization to controlled biomineralization when this became an advantage to their survival.
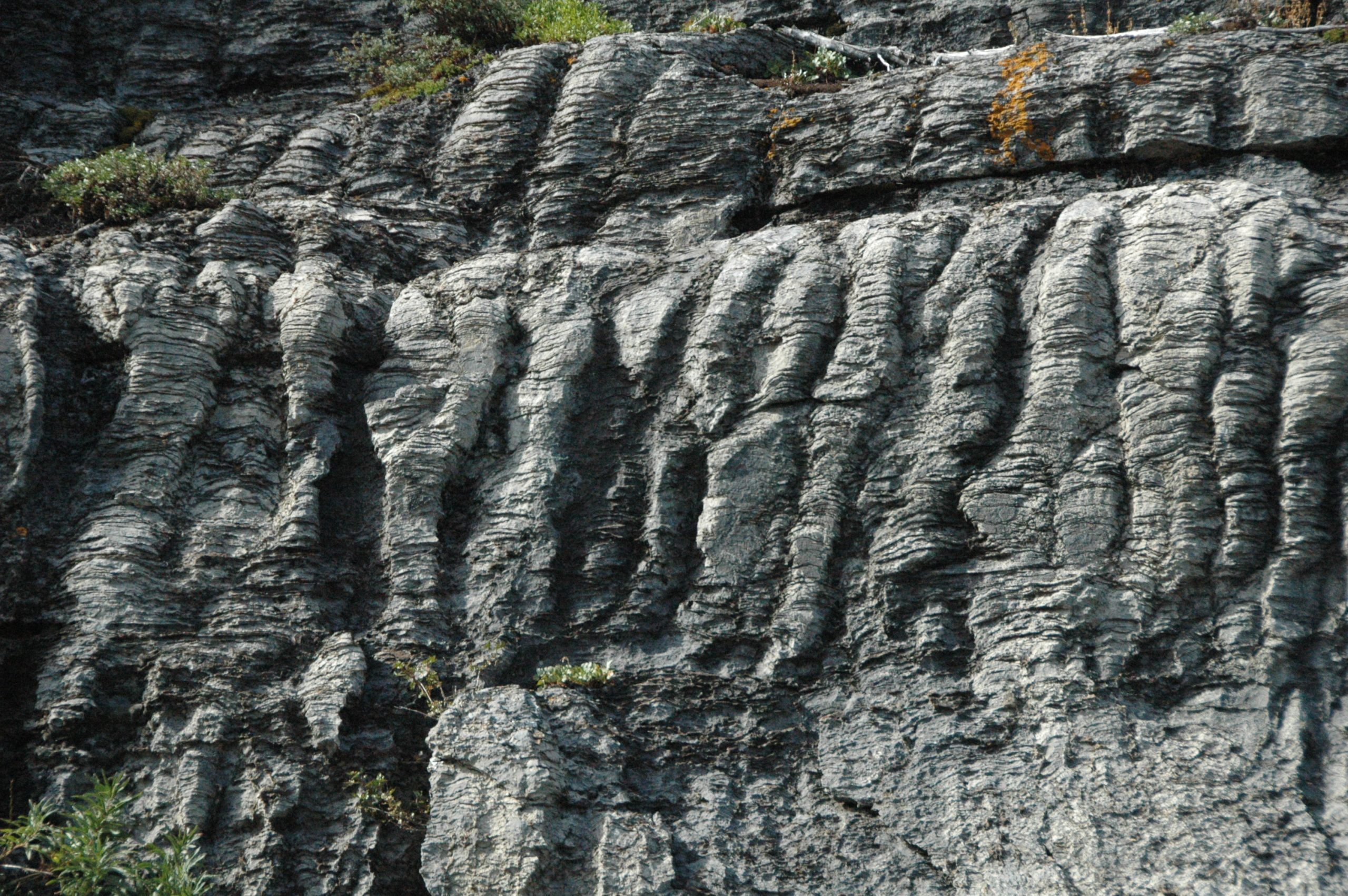
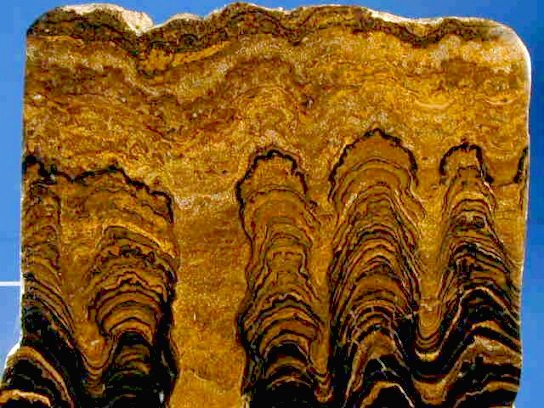
Stromatolites still exist at the present-day, but during later parts of Earth history, carbonate sediments have been increasingly deposited by eukaryotes, initially marine plants, and then (in the Phanerozoic Eon since about 540 Ma) by animals. An extraordinary variety of animals have built skeletons (including supports like those of corals and shells like those of molluscs and crustaceans) out of calcium carbonate.
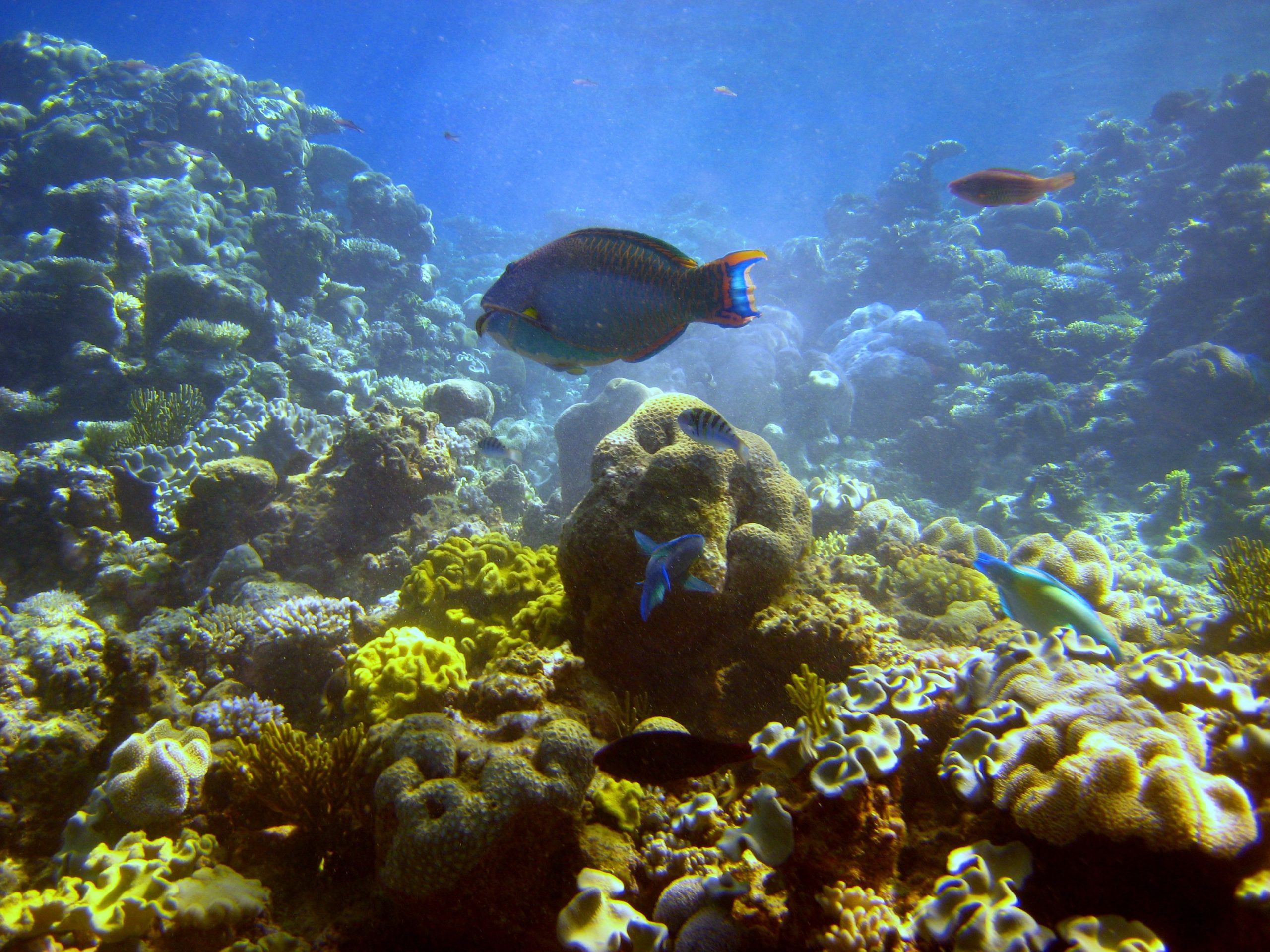
A connected mound of carbonate skeletons that is wave-resistant is known as a reef. At the present day corals (growing in symbiosis together with photosynthetic algae) are the main reef-builders, but several other groups of animals, including sponges and even molluscs have built reefs at different times in the Phanerozoic Eon – the last 540 Ma of Earth history.
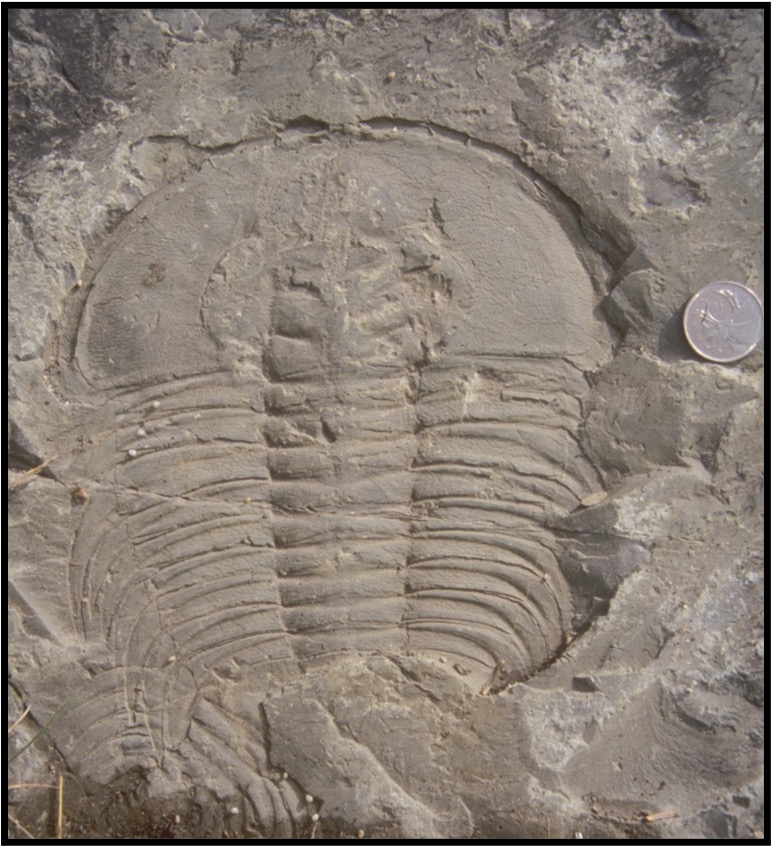
The above-mentioned types of carbonate deposit all occur in shallow-water marine environments, but carbonate sediment is also found in the deep ocean, where it is a product of planktonic organisms that live in the photic zone of the ocean but which sink to the bottom after death. Chalk is a soft pelagic limestone formed from the microscopic skeletons of single-celled plants called coccolithophores.
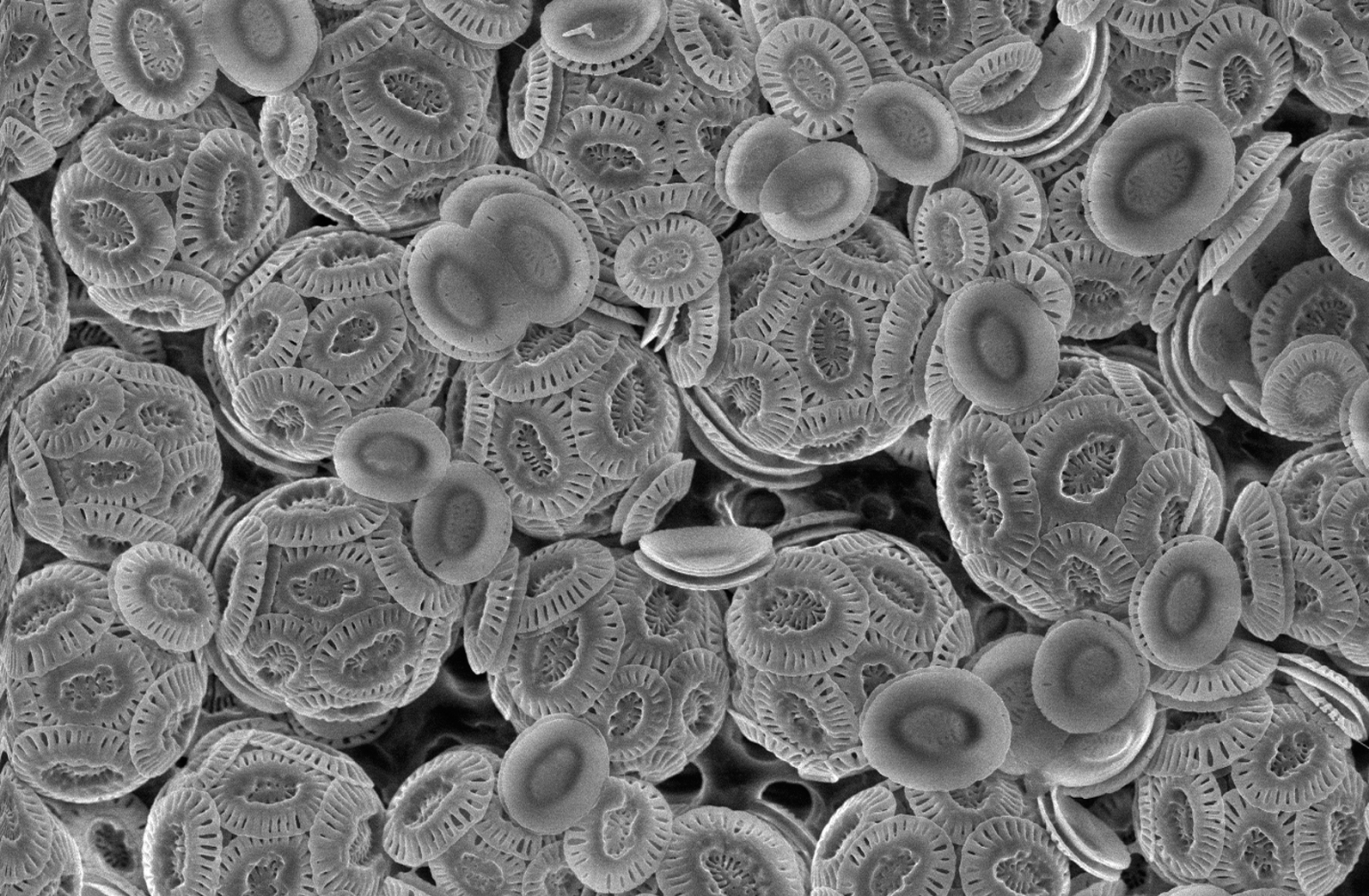
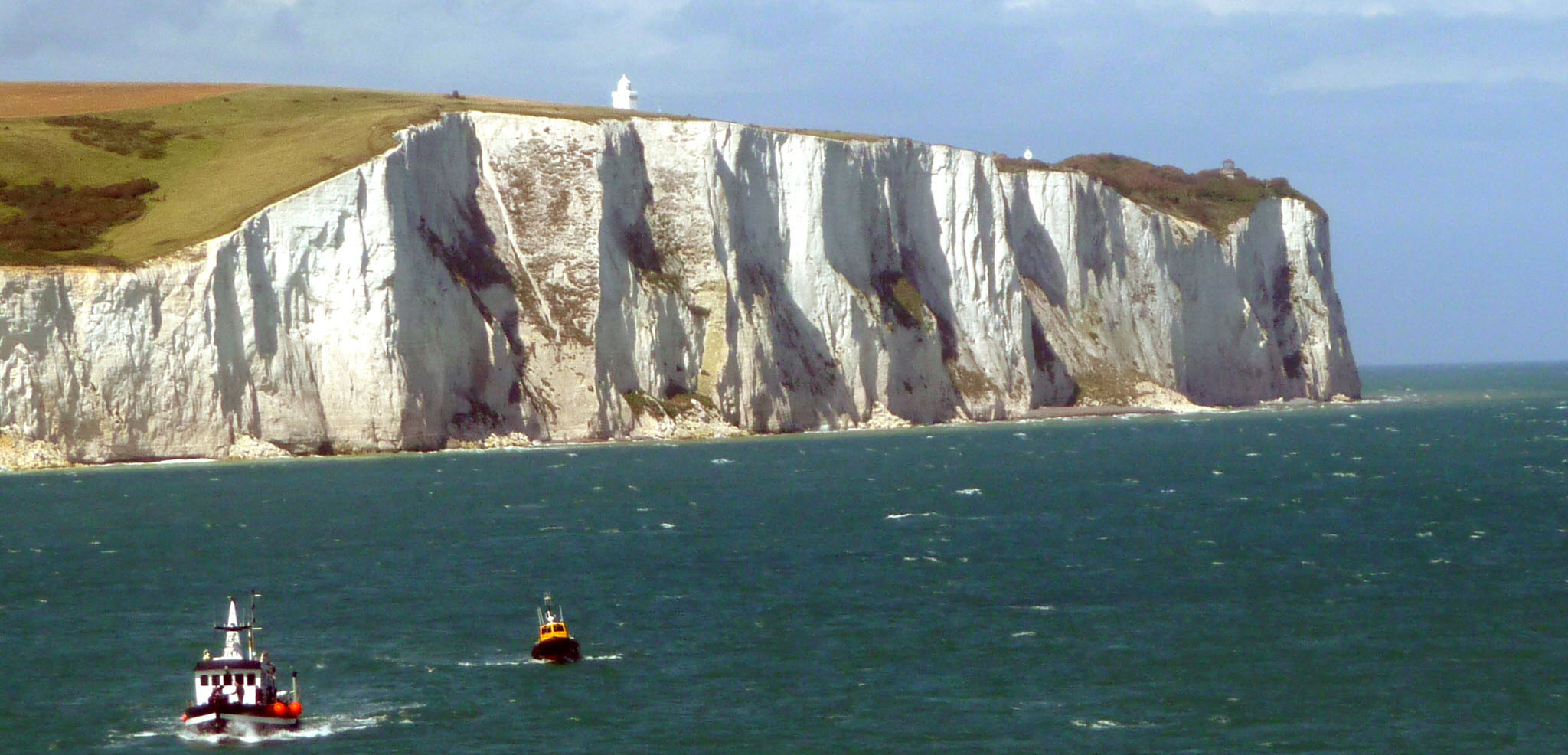
Silica biomineralization
In hot springs the hot (“hydrothermal“) fluids are often saturated in dissolved silica (SiO2) that precipitates as the water cools. Individual bacterial cells, and even entire communites, become encrusted in this silica, leading to the deposition of biologically induced silica.
Several groups of organisms have evolved the ability to extract silica from sea-water to produce skeletons. The diatoms are unicellular plants that are abundant in lakes and in cool ocean waters near the poles. Sediments deposited in these areas consist of diatomaceous ooze. In tropical waters, the most abundant silica extractors are Radiolaria, unicellular animals with beautiful silica skeletons. Deposits of Radiolaria on the ocean floor produce radiolarian ooze.
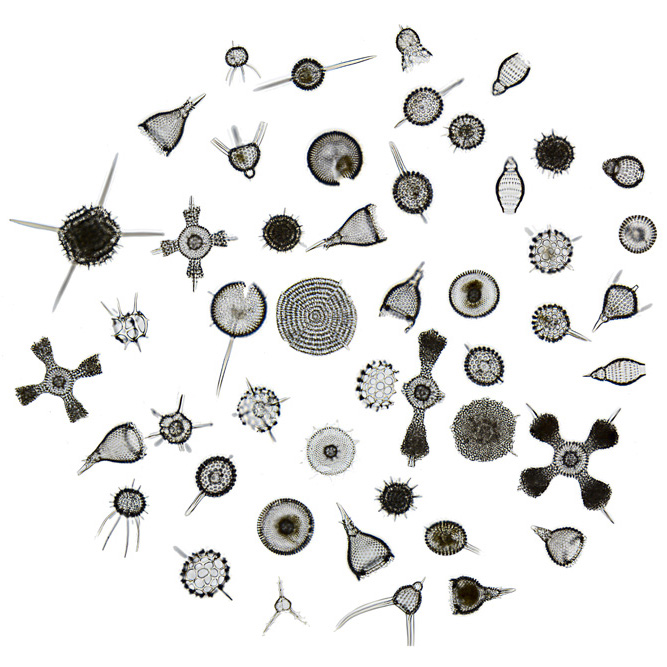
A third group of organisms that can control silica biomineralization are sponges: primitive metazoa that live on the sea floor. Sponges are rarely abundant enough to produce a direct deposit of silica, but their tiny silica needles (spicules) contribute to other kinds of sediment.
In all these examples, the silica produced by organisms is in the form of opal, a substance in which silicon and oxygen atoms are connected in a random tangle, comparable to that in glass. However, over millions of years, the silicon and oxygen atoms typically reorganize themselves into a regular crystal lattice, producing tiny grains of the mineral quartz, and forming a hard, fine-grained rock called chert, which was prized by early humans for making stone tools.
Phosphate biomineralization
Some organisms, notably vertebrates (including humans) concentrate phosphate ions (PO43-) in their skeletons, in teeth and scales, and in their excreted feces. Accumulations of vertebrate remains and of vertebrate feces may produce phosphate-rich sediment. Phosphate-rich sediment is much rarer than carbonate and silica-rich sediment, but where it does occur it may be mined by humans as a source of agricultural fertilizer.
The smallest unit of living things, including genetic material and cytoplasm surrounded by a cellular membrane.
Having a body comprising numerous cells separated by membranes from one another
An membrane-bounded body within eukaryotic cells that contains the genetic material in the form of DNA
Long, chain-like molecules (polymers) composed of linked units called nucleotides. Two major kinds of nucleic acids exist: DNA and RNA.
Deoxyribonucleic acid: A double-stranded nucleic acid that preserves genetic information
Complex fluid mixture fills the cells of prokaryotes; in eukaryotes it surrounds the organelles, nucleus, and ribosomes.
Structures within cells that accomplish important functions, typically relating to respiration, production of organic compounds, or reproduction
Organelles within the cells of eukaryotes are the site of respiration within eukaryotic cells
Organelles within plant cells that contain chlorophyll, and are responsible for photosynthesis in plants and photosynthetic microbes; chloroplasts are descended from free-living cyanobacteria.
consisting of a single biological cell
Having cells that contain a distinct nucleus surrounded by cytoplasm with organelles
A unicellular or multicellular organism with cells that contain a distinct nucleus surrounded by cytoplasm with organelles
Unicellular organisms whose cells lack a nucleus and complex organelles
Prokaryotic single-celled organisms; distinguished from Archaea by the chemistry of their cell membranes and ribosomes.
Single-celled prokaryotes capable of photosynthesis and the release of oxygen. Cyanobacteria were responsible for the original oxygenation of the Earth’s atmosphere.
A group of Prokaryotic single-celled organisms that diverged from Bacteria early in Earth history
A close association between two organisms, whereby both gain benefits from the relationship
Unicellular eukaryotes that are not plants, fungi, or animals; protists fall into multiple taxonomic groups
The most common process of cell division whereby both daughter cells acquire full copies of the parent cell’s genetic material
A stage in sexual reproduction of metazoa at which gametes fuse to form a cell with double the number of chromosomes.
The two-stage process of cell division in sexual reproduction, whereby four daughter cells are created each with half of a full set of chromosomes.
The carbon-nitrogen bonds between amino acids that build peptides and proteins.
Links within proteins between sulfur atoms in amino acids, that are important in defining protein shape and structure
Ribonucleic acid. A single-stranded nucleic acid that acts as to transfer genetic information in the production of proteins
A type of RNA responsible for the transfer of genetic information transcribed from DNA in the nucleus to ribosomes in the cytoplasm, where proteins are synthesised
Lipids involving a phosphate (PO₄)³⁻ group that gives one end of the molecule hydrophilic characteristics. Phospholipids are essential to the formation of cell membranes.
Barriers composed of phospholipids that are barriers within and around cells.
A complex carbohydrate polymer used by plants to store energy.
A polymeric carbohydrate found in plant del wals and bacterial biofilms; the most abundant organic substance in the biosphere
Elements or compounds essential for life processes.
Elements or compounds that, while essential for life functions, are only required in small quantities.
Nutrients which, when scarce, prevent cell division or growth from occurring, even in conditions where other nutrients are abundant of other nutrients.
Describes a substance that inhibits the growth, reproduction, or survival of an organism
a substance that inhibits the growth, reproduction, or survival of an organism
The flow of an element through not only the Biosphere and and other spheres of the Earth
A cycle describing the flow of the element Carbon through the Earth system.
Element 7. In the molecular form N2, the most abundant gas in Earth’s atmosphere.
The fifteenth element of the periodic table, essential to living things in the formation of membranes and the transfer of energy
Negatively charged ions formed by the oxidation of nitrogen; NO³⁻.
The transformation of atmospheric nitrogen into a form more usable by organisms.
A family of flowering plants (including peas and beans) capable of fixing nitrogen through symbiosis with nitrogen-fixing bacteria. Nodules within the legumes’ roots provide an anaerobic environment in which nitrogen fixation can occur.
The production of atmospheric nitrogen from ammonium ions by aerobic bacteria.
A gas composed of nitrogen and oxygen, produced as part of the nitrogen cycle, which acts as a greenhouse gas in the atmosphere; N₂O
Deposition by organisms of solid inorganic material obtained from dissolved material in their environment
Compound with the formula CaCO3, the main component of limestone and the shells of marine
animals
The production of reduced carbon compounds from water and carbon dioxide using light as an energy source, carried out by cyanobacteria, many algae, and plants.
The standard scale of a substance’s acidity or alkalinity; minus the logarithmic concentration of hydrogen ions; acids have low pH; alkalis (bases) have high pH.
An atom or group of atoms that has lost or gained one or more electrons, resulting in a net positive or negative electric charge
Finely layered (laminated) mounds or pillars of calcium carbonate deposited by cyanobacteria
A raised, wave-resistant mound or ridge on the sea-floor, formed mainly of calcium carbonate deposited by organisms such as corals and algae
Sedentary suspension feeders, closely related to sea anemones and jellyfish, which may build exoskeletons of calcium carbonate. They are major reef-builders in Earth’s oceans.
Primitive multicellular animals that lack true tissues and organs; most are filter-feeders
A phylum of invertebrates which typically produce shells composed of calcium carbonate; Mollusca include snails, clams, and octopus (a group that has secondarily lost the shell)
The current eon, following the Proterozoic, from about 539 Ma to the present day, characterized by abundant remains of muticellular organisms
Organisms that live floating or suspended in water
Soft limestone formed mainly from the calcite plates of unicellular planktonic plants called
coccolithophores.
Describes the open water column of the ocean; the environment away from the seafloor in which planktonic and nektonic organisms live.
Sedimentary rock composed mainly of calcium carbonate
Unicellular phytoplankton that secretes plates (coccoliths) of calcium carbonate to cover their bodies. Chalk is formed from these plates.
Hydrothermal describes groundwater that has been heated by geothermal energy
Silicon dioxide SiO₂
Photosynthetic, unicellular plankton that inhabit oceans and lakes. Diatoms are notable for their siliceous skeletons of opal.
A group of unicellular marine plankton that construct silica skeletons that settle to the ocean floor
Non crystalline silica, sometimes including a small proportion of water; SiO₂·nH₂O.
The regularly repeating three-dimensional arrangement of atoms and/or ions in a crystalline solid material such as a mineral
Silica SiO₂ in its most common mineral form.
A hard rock composed of very fine interlocking grains of silica SiO2.
Waste material expelled from an animal's gut