20 The Rock Cycle III: Metamorphism and Metamorphic Rock
This chapter is a near-final draft, requiring some additional figures and glossary entries.
Metamorphism
When buried in the Geosphere, rocks are affected by mechanical forces, and they may be heated to high temperature. These effects combine to recrystallize rocks, forming new minerals by chemical reactions that occur the solid state (without melting). This process is a little like cooking a cake in an oven (or in a pressure cooker), and is known as metamorphism.
Learning Oucomes
By the end of this section you should be able to:
- Distinguish the major causes metamorphism: heat, pressure, differential stress and deformation;
- Classify metamorphic rocks using different criteria;
- Summarize the rock cycle, including the formation of igneous, sedimentary, and metamorphic rocks.
Force, stress, and pressure
To understand metamorphism we need to know a little about the forces that act on rocks within the geosphere. Forces in physics are measured in Newtons (symbol N)
The effects of forces on rocks depend on how concentrated those forces are. Force concentration or force per unit area is called stress. Stress is measured in Pascals (Pa). 1 Pascal is 1 Newton per square metre. However, 1 Pascal is a very small stress: within the Geosphere we need to measure Megapascals (106 Pa) or Gigapascals (109 Pa).
In a fluid at rest, such as air or water, the stress is the same in all directions. This type of stress is pressure, also known as hydrostatic stress. All the forces act perpendicular to the surfaces of a body.
In a solid, the stress is not usually the same in all directions, so the analysis of stress is more complicated[1].
The state of stress in a solid can be divided into two parts. The mean stress, which is equivalent to pressure, is the part of the stress state which acts to change volume. Pressure increases steadily with depth within the Earth as it is mostly due to the weight of the overlying rocks. Increasing pressure encourages the formation of dense minerals, where the atoms are fitted together into a smaller volume.
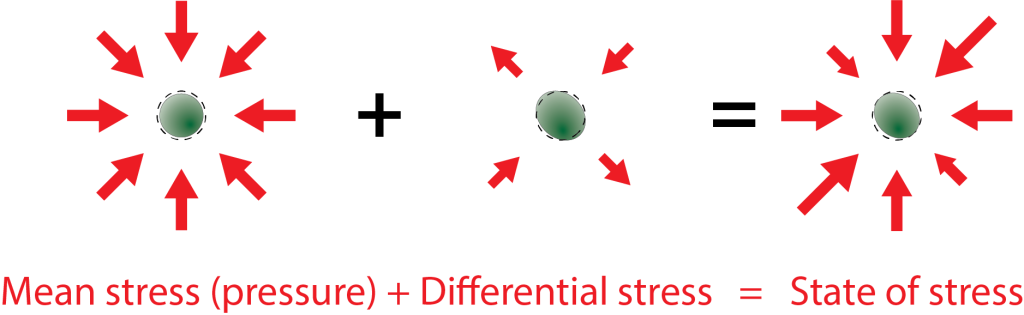
Differential stress[2] is the part of the stress that acts to change the shape of rocks by squeezing the rock in some directions and stretching it in others. The resulting change in shape, or deformation[3], can produce fabric — the alignment of minerals or other particles in a rock so that they follow a particular direction, like the grain in a piece of wood. Differential stress is very variable in the Geosphere but is most important near boundaries between tectonic plates.
Temperature
Temperature also increases with depth inside the Earth. On average, temperature rises with depth in the outermost part of the Geosphere at 25 to 30°C per kilometre, following a curve known as the geothermal gradient. However, the rate of temperature rise with depth is much more variable than the rise of pressure. Active volcanic areas like many plate boundaries typically show higher geothermal gradient than plate interiors. Temperature is of course particularly high adjacent to bodies of magma.
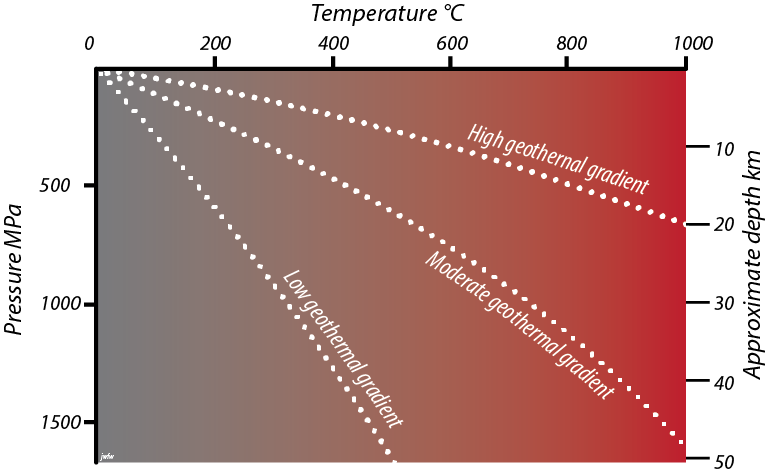
Increasing temperature has several effects on rocks. It acts to weaken rocks so that they deform more easily. In addition, high temperature encourages recrystallization so that grain size increases. Finally, minerals that are stable at the Earth’s surface, especially those like clay minerals that contain water, tend to become unstable at higher temperature, losing water and forming entirely new minerals.
Environments of metamorphism
Different combinations of temperature, pressure and deformation allow us to distinguish environments of metamorphism within the Geosphere.
Contact or thermal metamorphism is caused by contact with magma. The metamorphic effects are almost entirely the result of rise in temperature. Thermal metamorphism tends to increase the grain-size of rocks, and may eliminate fabric. Many of the mineral changes are similar to those that occur in clay that is fired in a kiln in the making of pottery.
Dynamic metamorphism is caused almost entirely by extreme deformation. It is not uncommon for rocks to be stretched and squeezed to fifty or more times their original length in dynamic metamorphism. This type of metamorphism produces very strong fabrics, and tends to reduce the grain-size.
Regional metamorphism involves temperature, pressure, and deformation in combination. Dynamic metamorphism is typical of the cores of mountain ranges produced by shortening of the Earth’s crust at convergent plate boundaries. Minerals produced by the regional metamorphism of certain kinds of rock (particularly mafic igneous rocks) provide sensitive indications of pressure and temperature. It is possible to divide a graph of pressure and temperature (a “P–T diagram”) into regions characterized by certain mineral assemblages, called metamorphic facies. For example, the blueschist facies is named for the presence of a distinctive blue chain silicate mineral called glaucophane that forms under conditions of high pressure but low temperature. It is indicative of subduction zones, where one plate is pulled beneath another.
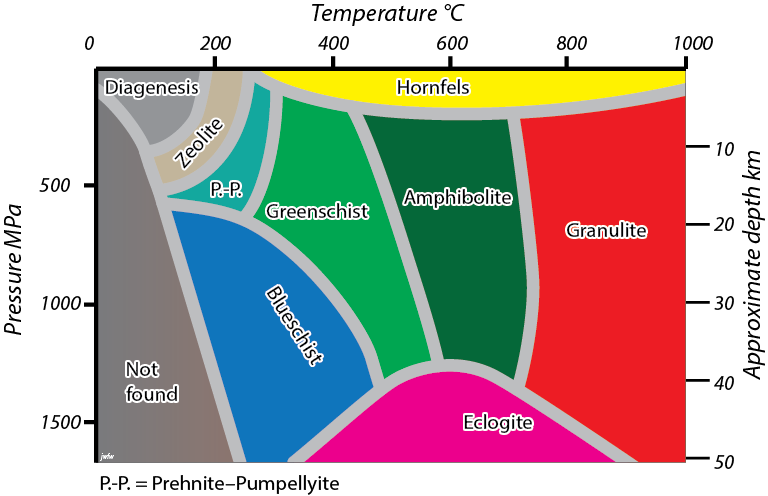
Metamorphic rocks
Naming metamorphic rocks
The names of metamorphic rocks can be confusing, because there are many different ways to name metamorphic rocks.
- Names based on fabric. Strong fabrics (alignment of particles within the rock) can be the most conspicuous feature of a metamorphic rock in hand sample, so rocks with strong fabric tend to be named according to their fabric. Slate, schist, and gneiss are examples of names based on fabric.
- Names based on composition. For rocks without fabric, it’s convenient to provide names based on mineral composition: Quartzite, marble, and greenstone are examples of such names.
- Names based on protolith. The protolith of a metamorphic rock is the type of rock prior to metamorphism. If the protolith is known, a metamorphic rock name can be made by attaching the prefix “meta-” to the protolith name, for example: metavolcanic rock; metasandstone.
- Finally, if we are able to interpret conditions of temperature, pressure, and deformation, it’s possible to name metamorphic rocks based on environment of formation. Examples are: hornfels, mylonite, blueschist.
As a result it’s possible for the same metamorphic rock to have four different names, all equally correct! For non-specialists, it’s best to name metamorphic rocks based on the observational characteristics of fabric and mineral composition, unless an interpretation is obvious (for example the rock is right next to an igneous intrusion).
Contact metamorphism
Contact or thermal metamorphism is metamorphism that occurs because of heat from nearby magma. A large intrusion, that carries a lot of heat, is typically surrounded by a halo or aureole of metamorphic rock, in which new minerals are developed.
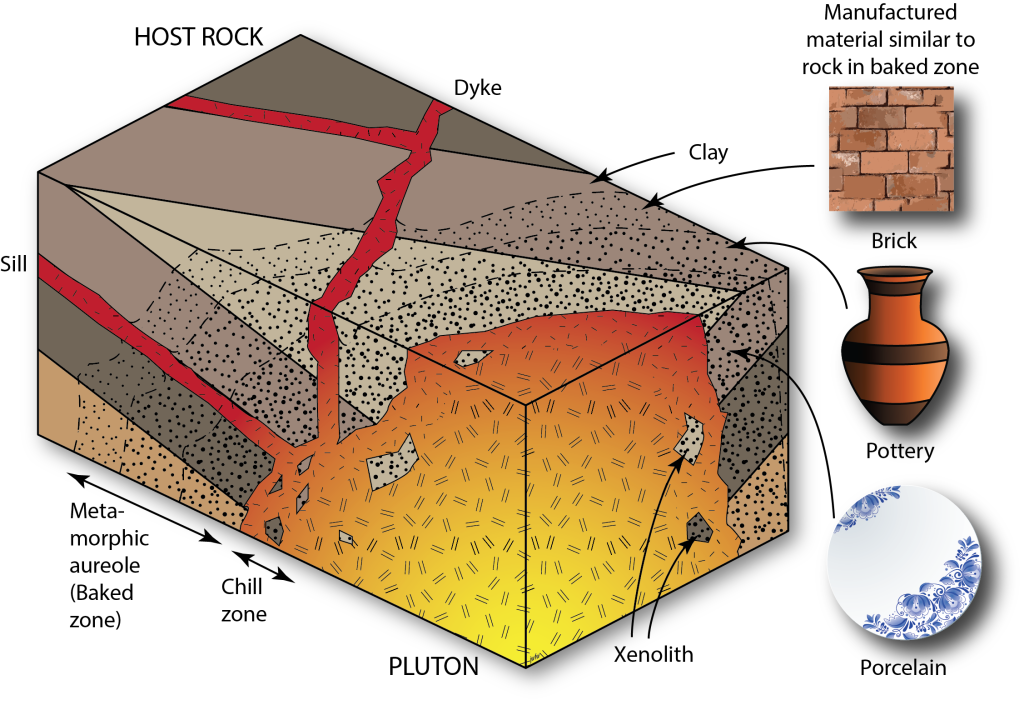
If the host rock of an intrusion happens to be mudstone then the changes that occur in the aureole are very close to those that occur when clay is fired in a kiln to make pottery. However, pottery is very fine-grained because firing usually only takes a day or two. A large intrusion may take thousands of years to cool, and metamorphic minerals often have time to grow large enough to be visible to the naked eye.
Metamorphic rocks formed in contact metamorphism have little fabric. In fact, if a rock like shale or slate is metamorphosed in contact metamorphism, the existing fabric is typically obliterated as minerals recrystallize without deformation.
A typical name for a hard metamorphic rock composed of silicate minerals, produced by contact metamorphism, is hornfels. (Note this word is singular, despite the final “s”. The plural would be “hornfelses”).
Very coarse grained rocks, with grains larger than about 2 mm, produced by thermal metamorphism of silicate protoliths, are described as granofels.
Dynamic metamorphism
Rocks flow in the solid state in response to differential stress. In shear zones the flow of rocks is extreme, and mineral grains may be stretched out into rods 50 or more times their original length. This has two effects on most rocks: it generates a strong fabric even if none existed before, and it causes mineral grains to break down and recrystallize to much finer grained material.
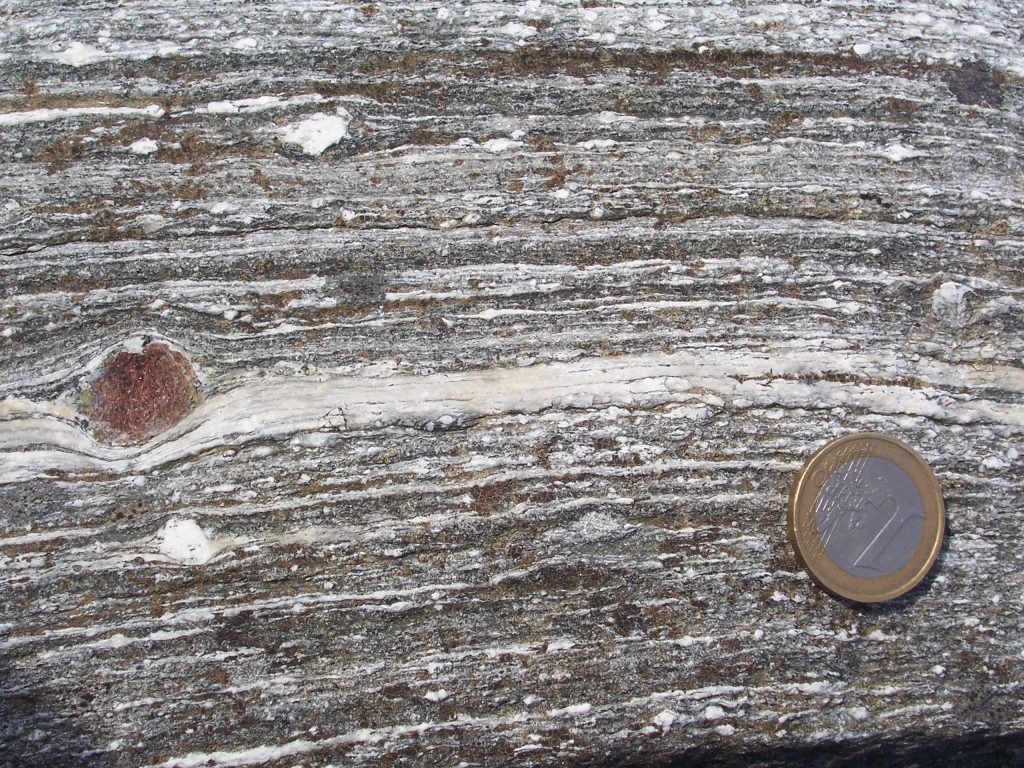
A rock that has had its grain-size reduced in this way is known as mylonite. Note that this term is an interpretation. Many mylonites can also be correctly described as schist. In other cases, where the mylonite lacks minerals like mica that confer a strong fabric, mylonites can also sometimes be called marble (if carbonate-dominated) or quartzite (if mainly quartz).
Regional metamorphism
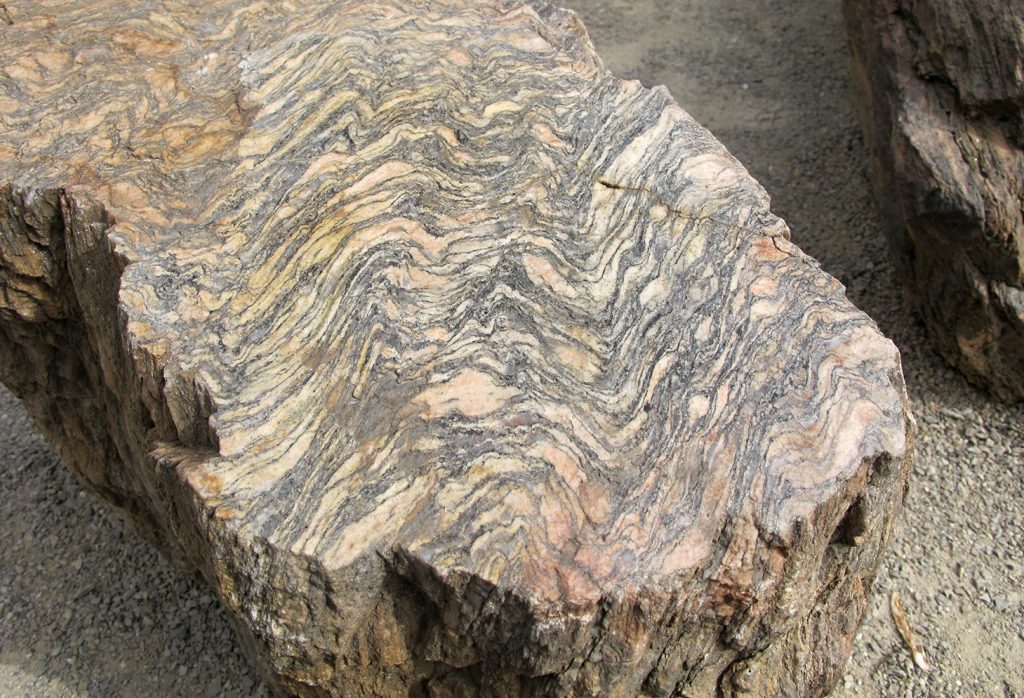
Rocks affected by regional metamporphism typically show the effects of both high temperature and high pressure, usually accompanied by deformation caused by differential stress. Regional metamporphism is typical of the interiors of mountain belts, or orogens, developed where plates move towards each other. (These environments are described in more detail in the section on plate tectonics.)
Regional metamorphism typically produces new minerals. The exact minerals formed depend on both the composition of the protolith and the conditions of metamorphism — the temperature and pressure.
Regional metamorphic rocks typically display strong fabrics, and other structures like folded layers, that are indications of deformation or flow produced by differential stress.
Regional metamorphic rocks provide important information on past plate movements and tectonic environments.
Regional metamorphic rocks with strong fabric are typically given names based on the type of fabric. If fabric is weak or absent, regional metamorphic rocks are typically named based on the overall rock composition and mineralogy.
Rocks with strong metamorphic fabric
Slate
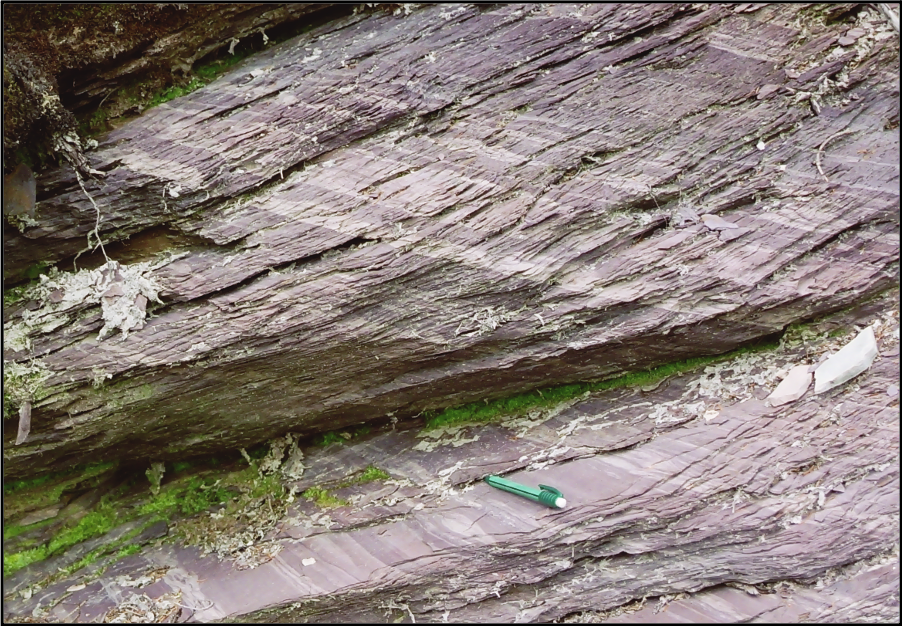
Fine grained sedimentary rocks that have been subject to regional metamorphism at relatively low metamorphic temperature (low grade) typically show a distinctive fabric called cleavage. Cleavage is produced when sheet silicate minerals, typically flake-shaped, are re-oriented by deformation. The flakes are typically perpendicular (at 90°) to the shortening direction. Cleavage resembles the fissility that is characteristic of shale, but unlike fissility, cleavage is not typically parallel to the layers in a metasedimentary rock.
You may have noticed that the same word, cleavage, is used to describe a property of individual minerals and whole rocks. Although the two uses both refer to easy splitting, the cleavage of minerals is produced by the arrangement of atoms within a crystal, whereas the cleavage of metamorphic rocks is produced by the alignment of mineral grains within a rock as a whole.
Fine-grained metasedimentary rock that displays cleavage is known as slate. Slate has been widely used as a roofing material in areas where it is abundant. Slate can also form by the metamorphism of fine-grained tuff: metamorphosed pyroclastic igneous rock.
Schist
Higher metamorphic temperatures lead to grain-growth. A coarse-grained equivalent of slate, in which mica or other oriented mineral grains are clearly visible, is called schist. Although schists appear very different from slates in hand sample, really the difference is just one of grain size. In fact, the boundary between slate and schist is gradational. Many texts mention a third, intermediate category of metamorphic rock with strong fabric, phyllite. However, the term phyllite has been somewhat inconsistently used, and in our course we just recognize slate (with grains too small to be separated without magnification) and schist (with visible grains defining the fabric).
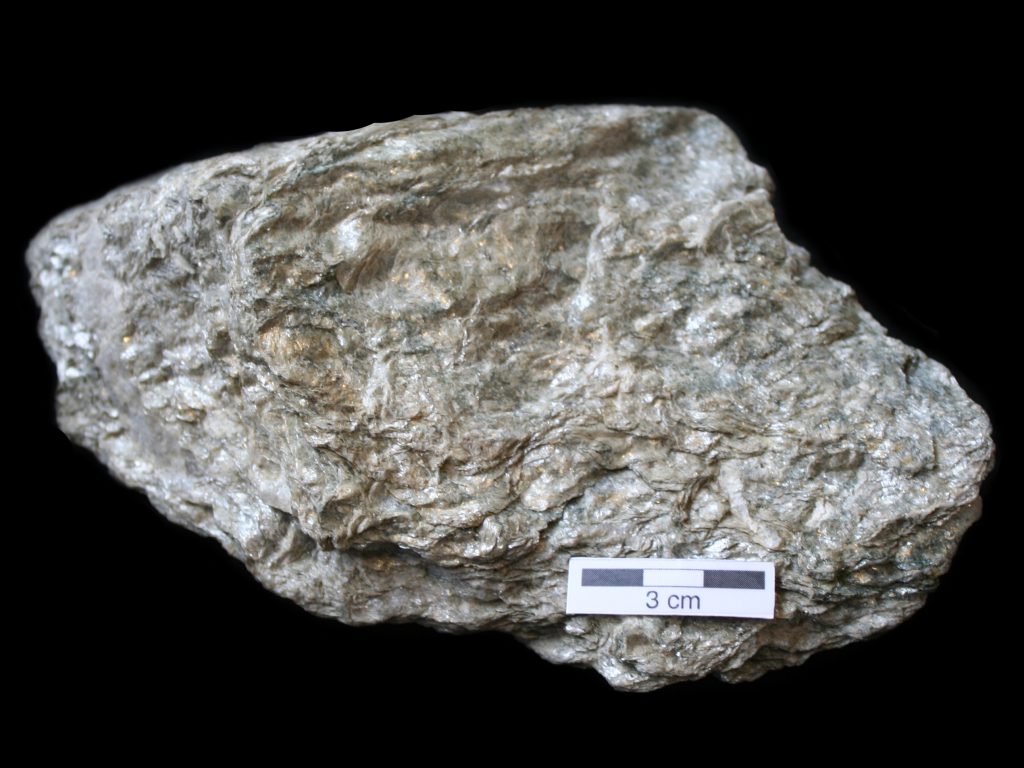
In greater detail:
Gneiss:
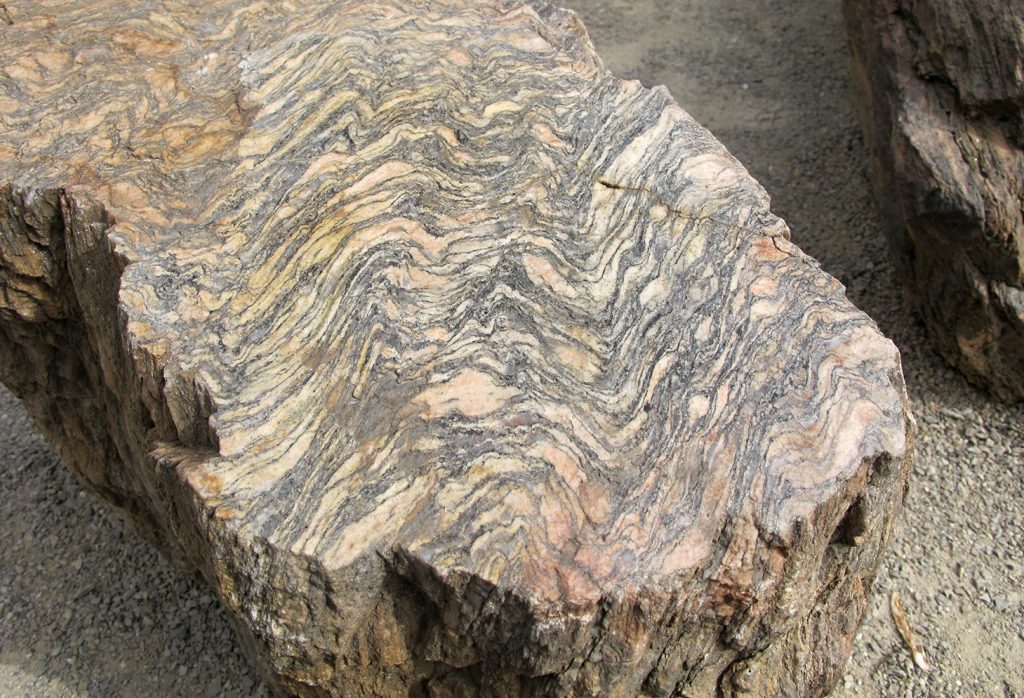
The highest temperature (highest grade) regional metamorphic rocks tend to show distinct banding, in addition to the alignment of mineral grains. In some circumstances this banding is due to original layers present in a sedimentary protolith. More commonly, however, banded metamorphic rocks are formed by pressure solution which removed quartz from fabric planes as the rock is deformed, or by partial melting which developed layers or patches of magma, usually felsic, in a rock which was metamorphosed at high temperature. Layered high-grade regional metamorphic rocks are called gneiss (pronounced “nice”). Gneisses that have formed by partial melting can also be termed migmatites. Note that once again a rock may correctly be given two different names, one of which (gneiss) is purely descriptive and the other of which (migmatite) implies an interpretation of the rock as a product of partial melting.
Metamorphic rocks without strong fabric
Quartzite
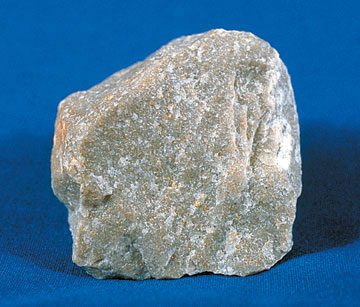
When fabric is absent or weak, metamorphic rocks may be named according to the minerals present. Quartzite is a metamorphic[4] rock in which quartz is the most abundant mineral. Quartzite is most commonly formed by the metamorphism of sandstone, although quartzite may have other quartz-rich protoliths like chert. Quartzite is distinguished from sandstone because, following metamorphic recrystallization, quartz grains interlock with one another, whereas in a sandstone the sand grains rest against one another as they were deposited. When quartzite is broken, it’s normal for the fractures to pass through the quartz grains, giving the surface an appearance like frosted glass; sandstones commonly break around the grains, so that broken surfaces appear sandy.
Marble
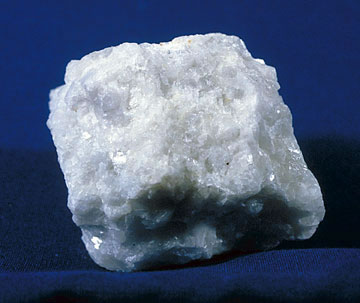
Calcite is also stable over a wide range of conditions, with the result that metamorphosed pure limestone typically consists of calcite like its protolith. However, features like fossils and other original sedimentary grains are typically obliterated, producing a rock, marble, that is typically made of coarse interlocking crystals. Because calcite is a relatively soft mineral, marble has been much use for carving and for ornamental building stone.

Greenstone
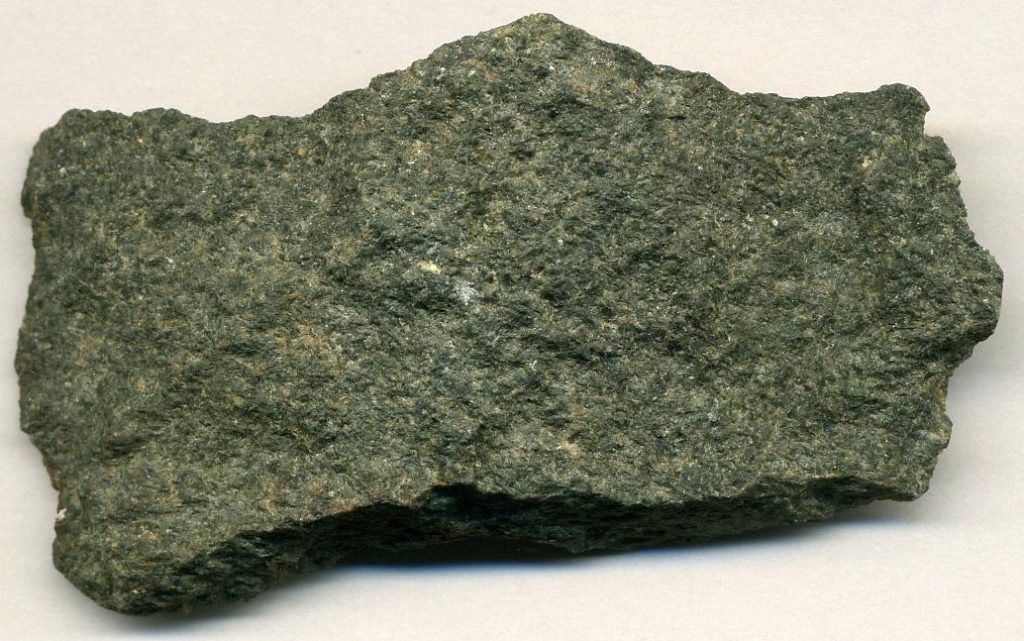
Mafic volcanic rock is typically composed of minerals that are stable at high temperatures (1000°C or more) at which mafic magma solidifies. Metamorphism therefore may convert these to lower-temperature ferro-magnesian minerals. These rocks are typically dark in colour, though they tend to be slightly more green than their protoliths. Metamorphosed mafic igneous rocks without strong fabric may be termed greenstone.
The rock cycle: summary
In this section we recap the major processes that we have encountered in the study of igneous, sedimentary, and metamorphic rocks to build a picture of the cycling of solid materials, mainly silicate minerals, through the Earth’s crust in the rock cycle. Over time, the rock cycle is what has given the Earth its distinctive and varied crust.
Partial melting is a logical start for a description of the rock cycle, because it is the process most responsible for the formation of crustal rocks from the Earth’s original mantle. Magma is a mobile mixture of liquid and solid material formed by partial melting. Because most silicates expand as they melt (the opposite behaviour to water), becoming less dense, magma typically rises within the Geosphere. Magma cools either within the Geosphere or when erupted onto the surface, crystallizing to produce igneous rock. Slow cooling within the Geosphere produces coarse-grained intrusive rock; fast cooling at the surface produces fine-grained extrusive or volcanic rock.
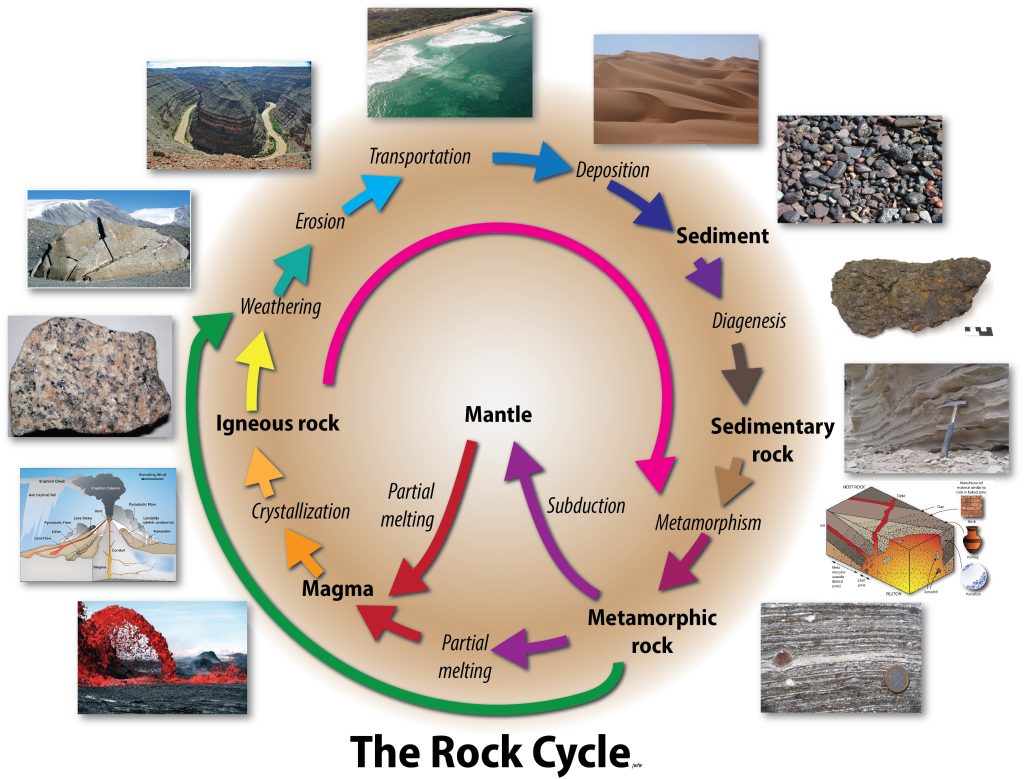
When exposed at the surface of the Geosphere to the actions of the Atmosphere, Hydrosphere, and Biosphere, rocks undergo physical and chemical changes called weathering. Weathered material that remains in place at the surface of the Geosphere is called regolith; when mixed with organic matter it is called soil. However, weathered material is in many circumstances removed from the location of weathering by erosion. The eroded material may be transported by wind, water, ice, living things, or simply by gravity, before being deposited at a new location as sediment. Sediment may undergo lithification (part of diagenesis) to form sedimentary rock.
Either sedimentary or ignous rock may be subject to changes of temperature and pressure, and to deformation within the crust. This causes recrystallization in the solid state, or metamorphism, leading to the formation of metamorphic rock. At very high temperature, metamorphism may lead to partial melting, which returns us to the start of the rock cycle.
Our rock cycle includes the main groups of crustal rocks — igneous, sedimentary, and metamorphic — but is not quite complete. It’s common for igneous rock to be metamorphosed, so we need a shortcut going directly from igneous rocks to metamorphism, without passing through sediment. Also, it’s common for metamorphic rocks to be weathered, eroded, and converted to sediment, so we need an arrow directly from metamorphic rocks to sedimentary processes without passing through igneous rock. Is there a third shortcut from sedimentary directly to igneous rocks? Probably not, because it’s impossible to melt sedimentary rocks without metamorphosing them first!
Finally, we started by considering the processes (partial melting) that forms new crust from mantle rocks. Is there a process that returns crust to the mantle? Yes there is: it’s the process of subduction, described in the section on tectonics, where one plate of lithosphere dives back into the deeper mantle, carrying its layer of crust with it. Over very long periods of time, it’s likely that slightly less crust is subducted than is generated by partial melting in the mantle, so the volume of crust has grown during the 4.6 Ga history of the Earth.
- For physicists, stress is a tensor quantity, not a scalar. In some texts, the force concentration vector on a single surface is distinguished as traction. We here use stress for both quantities, distinguishing the tensor as state of stress where necessary. ↵
- For physicists and engineers: For simplicity, we here use the simple scalar concept of differential stress, representing the difference in magnitude between the maximum and minimum principal stresses, instead of the more technical concept of deviatoric stress, a tensor quantity that more completely represents the part of the stress that acts to change shape. ↵
- Many introductory texts in Earth science imply that stress produces fabric, and therefore that stresses can be simply interpreted from fabric. This is incorrect, because if the rock materials rotated during ductile deformation the strain axes may no longer be parallel to any stress that operated in the history of the rock; also, if the rock is cold and strong a high stress may produce very little fabric, whereas if the rock is hot and weak a strong fabric may be produced by low differential stress. Therefore neither the amount nor the orientation of fabric gives direct insight on stress. Fabric is much more closely related to strain than to stress. ↵
- Quartz as a mineral is stable over a huge range of temperatures and pressures, with the result that it is sometimes difficult to distinguish a sedimentary sandstone that has been cemented by quartz from a metamorphic quartzite. Quartz-cemented sandstones are sometimes even called orthoquartzite, whereas true metamorphic quartzite can be called metaquartzite if there is any ambiguity. ↵