23 Tectonic Settings and Earth Resources
This chapter is pre-review version.
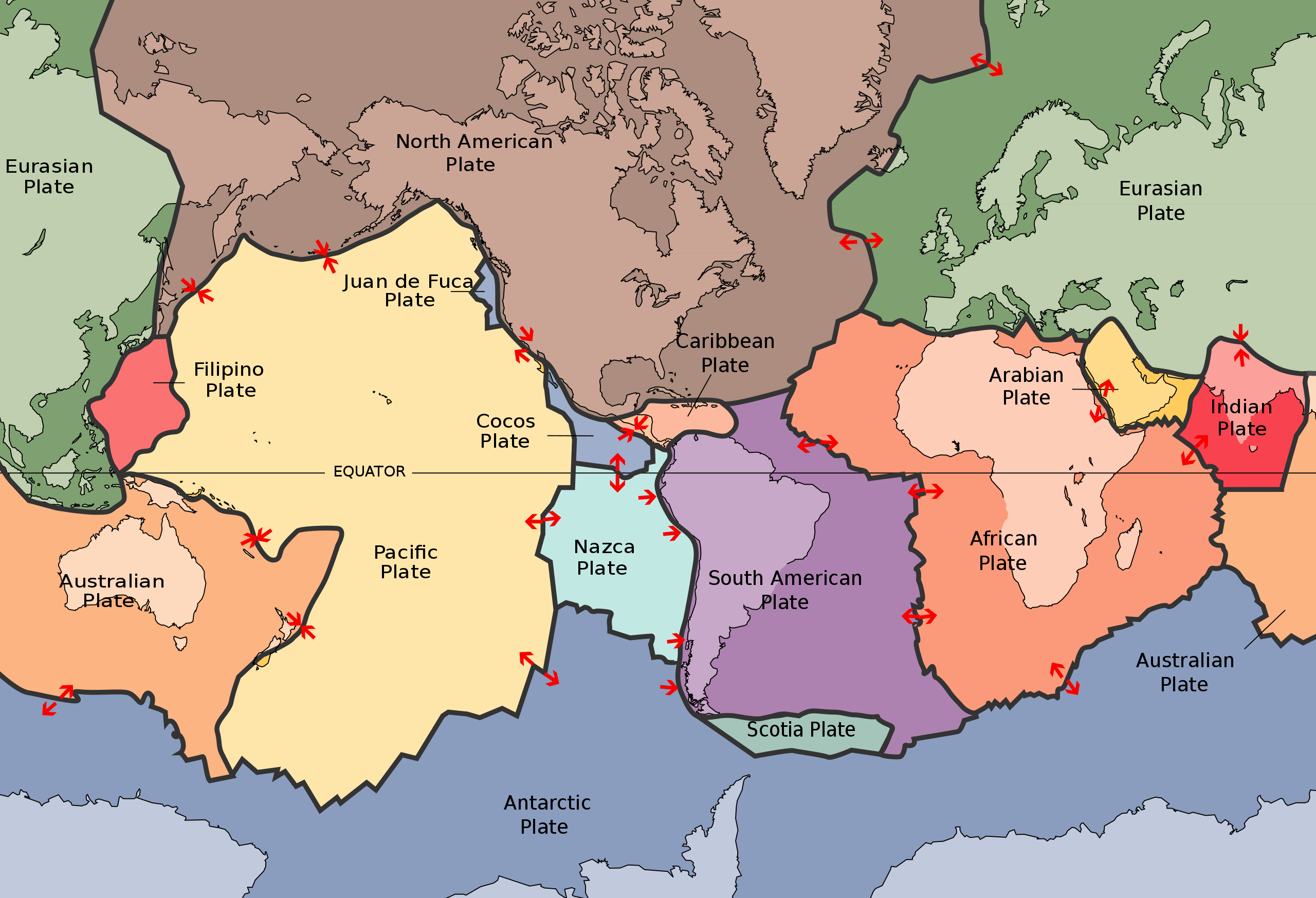
In this chapter we look at the major parts of the tectonic system, to see what types of phenomena occur in each. We pay particular attention to those Geosphere features – minerals and energy resources – that have been exploited by our own species, Homo sapiens over the course of history. We break down tectonic settings into those associated with plates that move apart (divergent or extensional settings), those associated with plates that move past each other without converging or diverging (transcurrent or strike-slip settings), and those where plates move towards each other (convergent settings).
Learning Outcomes
By the end of this chapter you should be able to
- Differentiate between renewable and nonrenewable resources, and reserves
- Identify major tectonic environments in the Earth’s crust and describe their characteristics
- Match major resources used by humans to their tectonic settings
Before plunging in to these tectonic environments, we will take a quick look at how resources are categorized when they are useful and therefore have economic value to humans.
Earth resources
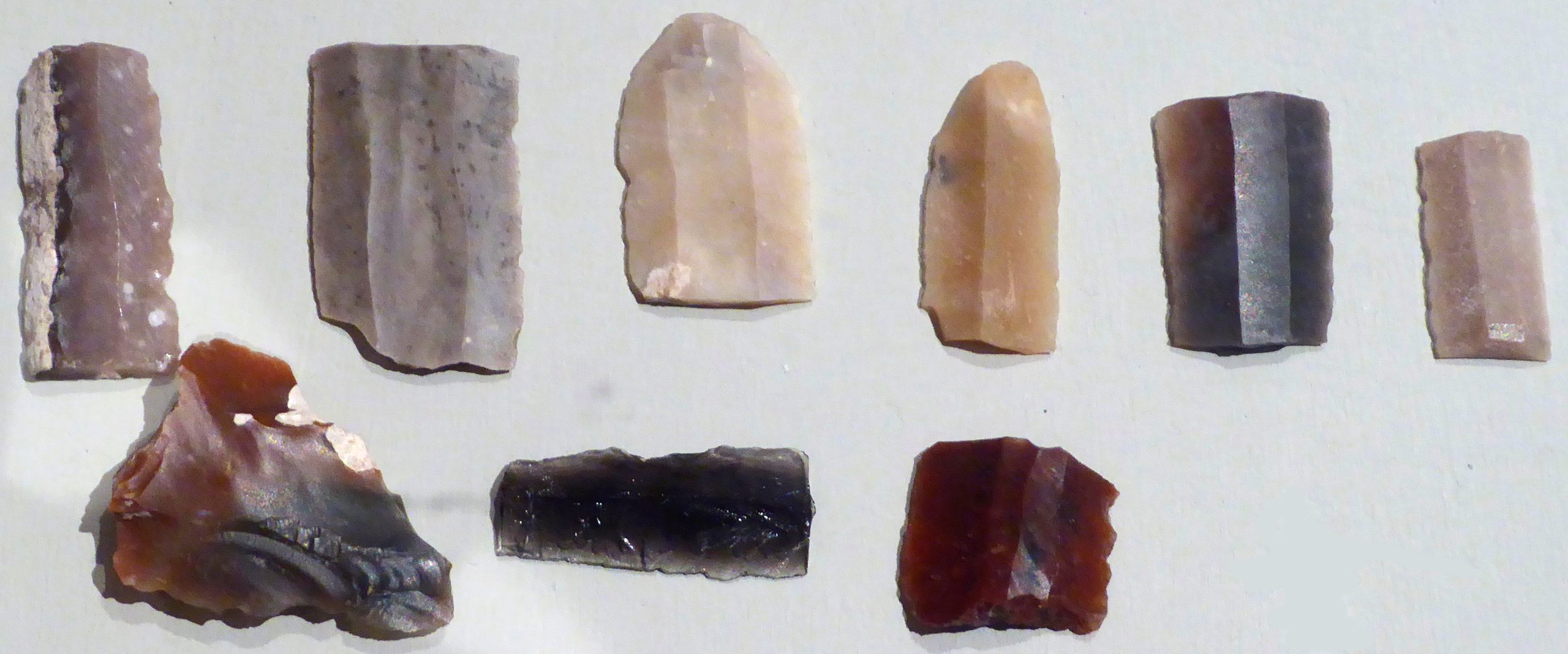
Most organisms draw energy and matter, known as resources, from their immediate environments in order to support their living processes. The human species, Homo sapiens, has used materials from the Earth to an unparallelled extent, seeking out and creating systems for the extraction of materials and energy from the spheres of the Earth in support of its activities. In addition to energy, and water, humans use many solid materials, some of which are sourced from the Biosphere (e.g. wood), but many of which are obtained from the solid Earth, the Geosphere, by mining. This is true of all the materials used in modern technology, including the computing device on which you are most likely reading these words.
In this section we will examine the major geographic divisions of the Earth’s tectonic system that result from plate tectonics, and show how particular resources are concentrated in certain environments. In a separate section we will focus on the impacts of the byproducts of those resources, particularly those associated with energy production, impact the Earth’s climate system. Resources and their impacts are a huge topic. As in many sections of this overview, we will only have time to scratch the surface of major issues.
Classification of resources
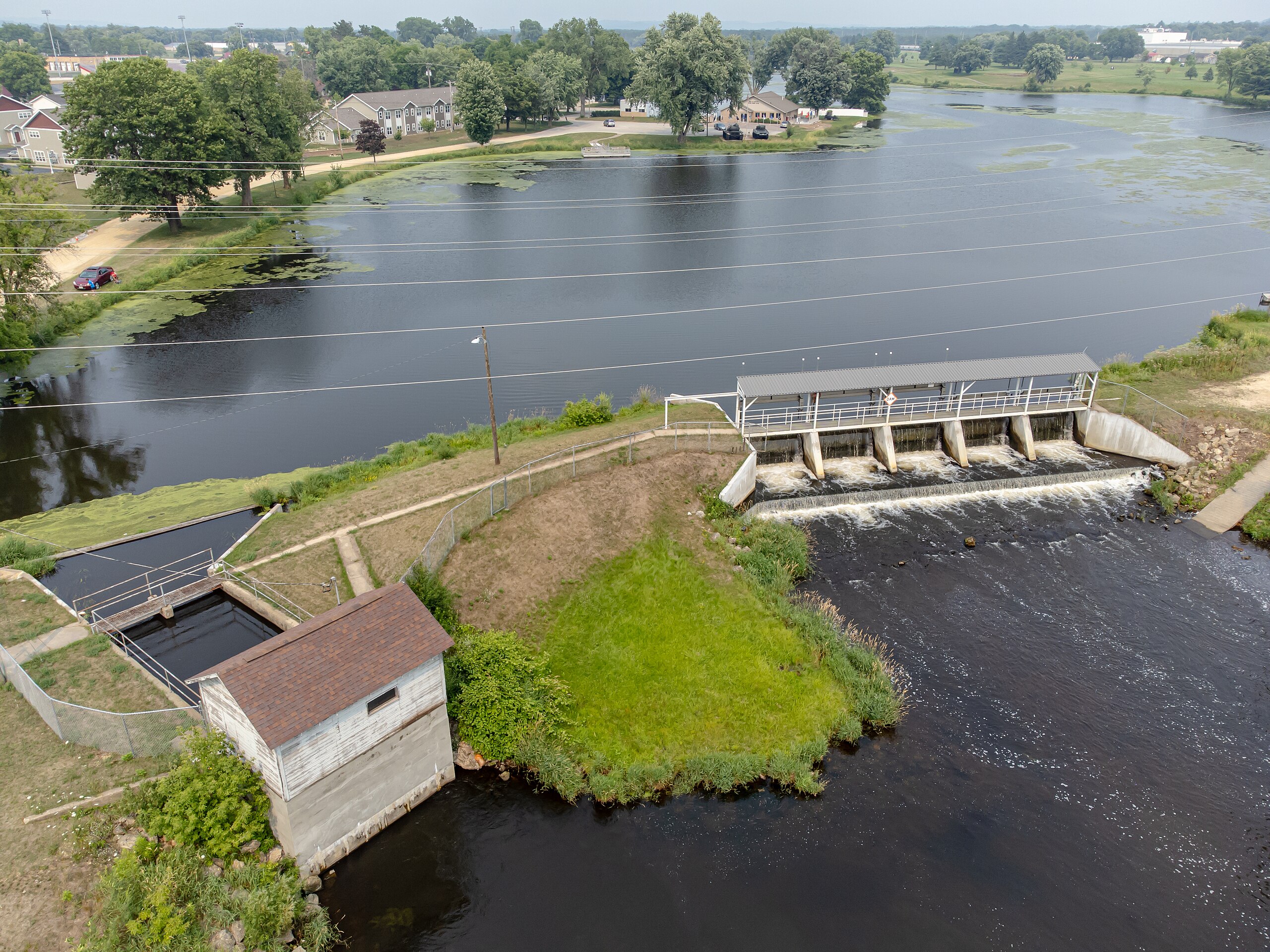
- Most resources that humans obtain from the Geosphere are used at a higher rate than they are returned. Examples include carbon-based energy, used by humans at a rate of about 16 TW, but returned to the Geosphere by photosynthesizing plants at a much slower rate (estimated at about 0.2 TW). Such resources are known as non-renewable.
- Some resources from atmosphere, hydrosphere, or biosphere are used by humans at rates less than the fluxes in their natural cycles. Such resources are said to be renewable. Examples include surface water, some groundwater, food crops, wood, wind power, and solar power.
Although public concern is often directed at non-renewable resources, it’s important to note that both renewable and non-renewable resources can have major impacts on the environment at the surface of the Earth.
Resources and reserves
Most elements of the periodic table are present everywhere in the Earth’s crust, though in many cases a given element is present in very small concentrations in most places. The concentration of a mined element is known as its grade. To be practically or economically useable by humans, elements have to have been concentrated by natural processes before they can be used. For example, gold is present in tiny amounts (1–3 parts per billion) in most rocks of the Earth’s continental crust. To be worth extracting, even at the high price commanded by gold, it has to have been first concentrated by natural processes, to a grade about 2,000 times its average crustal abundance. The rarity of such circumstances helps to determine both the availability and the price of gold.
Metal | Typical Background Level | Typical Economic Grade | Concentration Factor |
---|---|---|---|
Copper Cu | 40 ppm | 10,000 ppm (1%) | 250 times |
Gold Au | 0.003 ppm | 6 ppm (0.006%) | 2,000 times |
Lead Pb | 10 ppm | 50,000 ppm (5% | 5,000 times |
Molybdenum Mo | 1 ppm | 1,000 ppm (0.1%) | 1,000 times |
Nickel Ni | 25 ppm | 20,000 ppm (2%) | 800 times |
Silver Ag | 0.1 ppm | 1,000 ppm (0.1%) | 10,000 times |
Uranium U | 2 ppm | 10,000 ppm (1%) | 5,000 times |
Zinc Zn | 50 ppm | 50,000 ppm (5%) | 1,000 times |
For non-renewable resources, economic estimates are made in 2 categories: resources and reserves.
- Resources: naturally occurring accumulations of useful organic or inorganic material in concentrations and amounts that make extraction currently or potentially feasible.
- Reserves: resources that can be exploited economically (under present conditions).
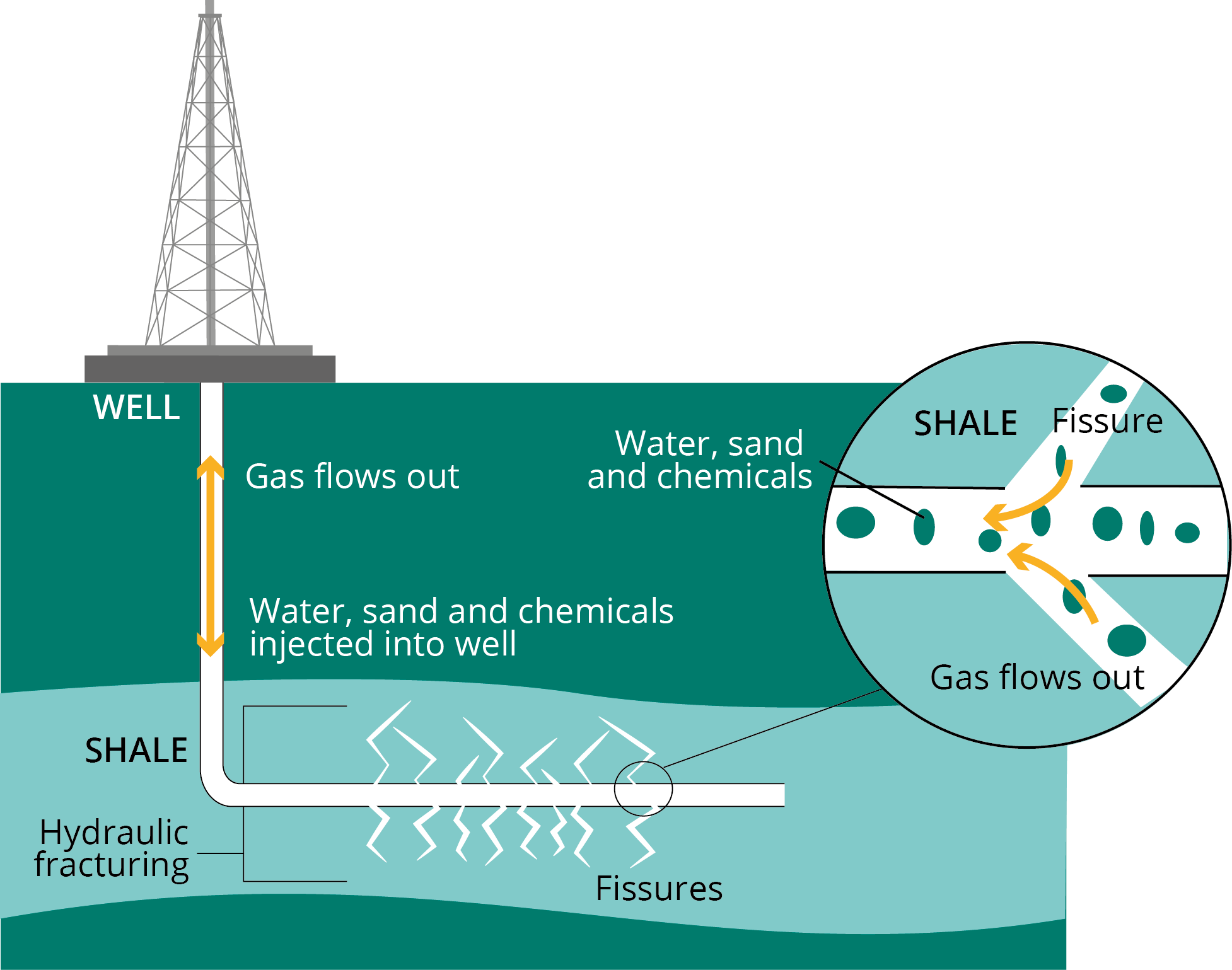
Notice that both categories are to some extent “moving targets”. Resources are estimated amounts and may change with new discoveries. Reserves are typically of more interest to economists and those who develop public policy, but what can be exploited depends on the current price (which may vary) and on technological developments (which may a previously impractical resource into a practical one). For example, for many years the hydrocarbons trapped in impermeable layers of mudstone were not regarded as reserves because they could not be made to flow to the Earth’s surface. The development of horizontal drilling and hydraulic fracturing (“fracking”) made many previously uneconomic resources of oil shale and shale gas into economic reserves, provoking controversies while previously rural environments saw rapidly increased industrial activity.
Extensional environments: plates moving apart
Spreading centres

Plates move apart at spreading centres, typically marked by mid-ocean ridges. At the spreeading centre, asthenosphere rises adiabatically (without loss of heat) into the space between the plates. As it rises, it experiences a fall in pressure which typically causes partial melting. Up to 30% of the mantle peridotite may be melted. The magma, being less dense than the surrounding peridotite, percolates up toward the surface, collecting together and solidifying to form new crust, made of gabbro, mafic dykes and pillow lavas.
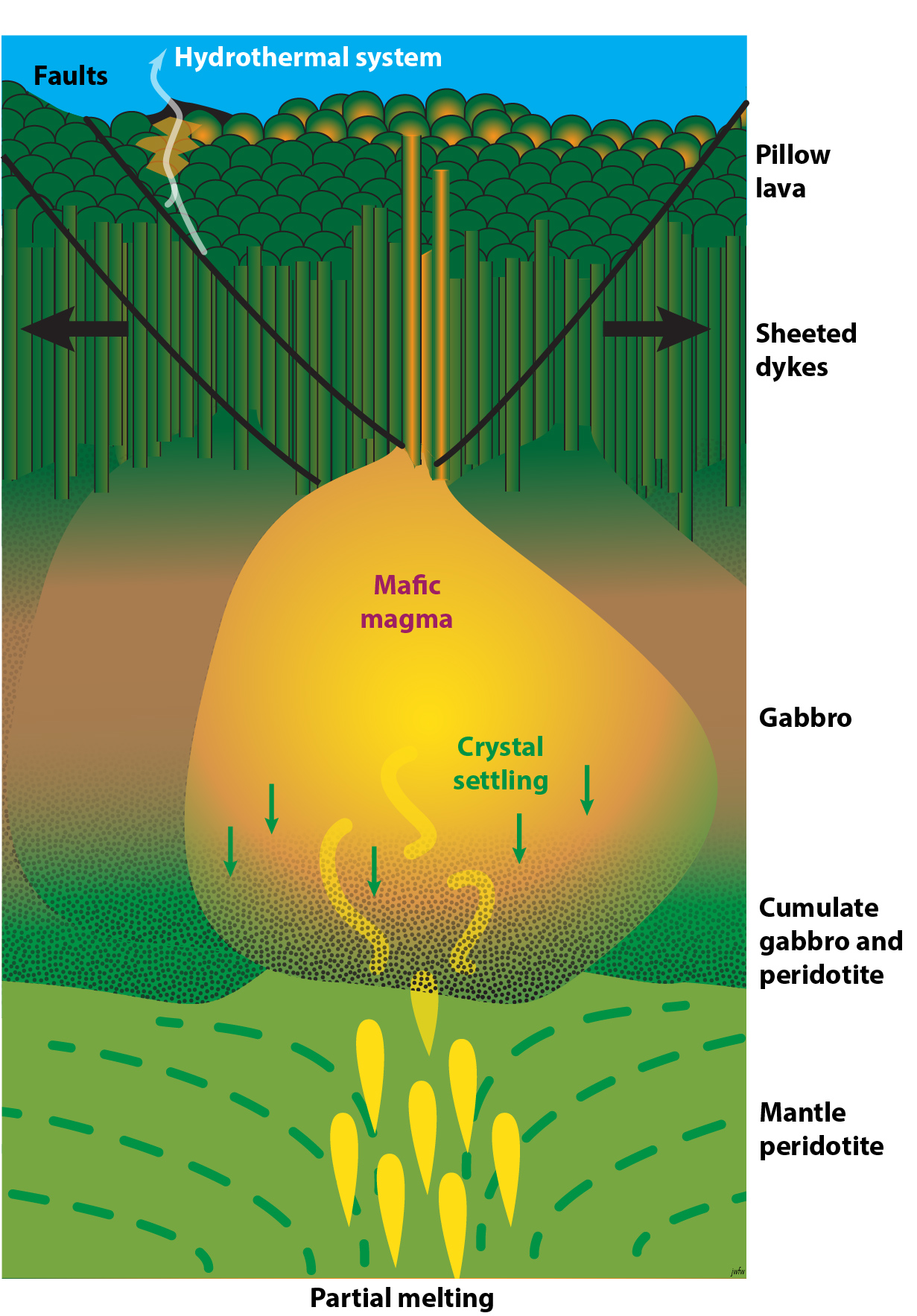
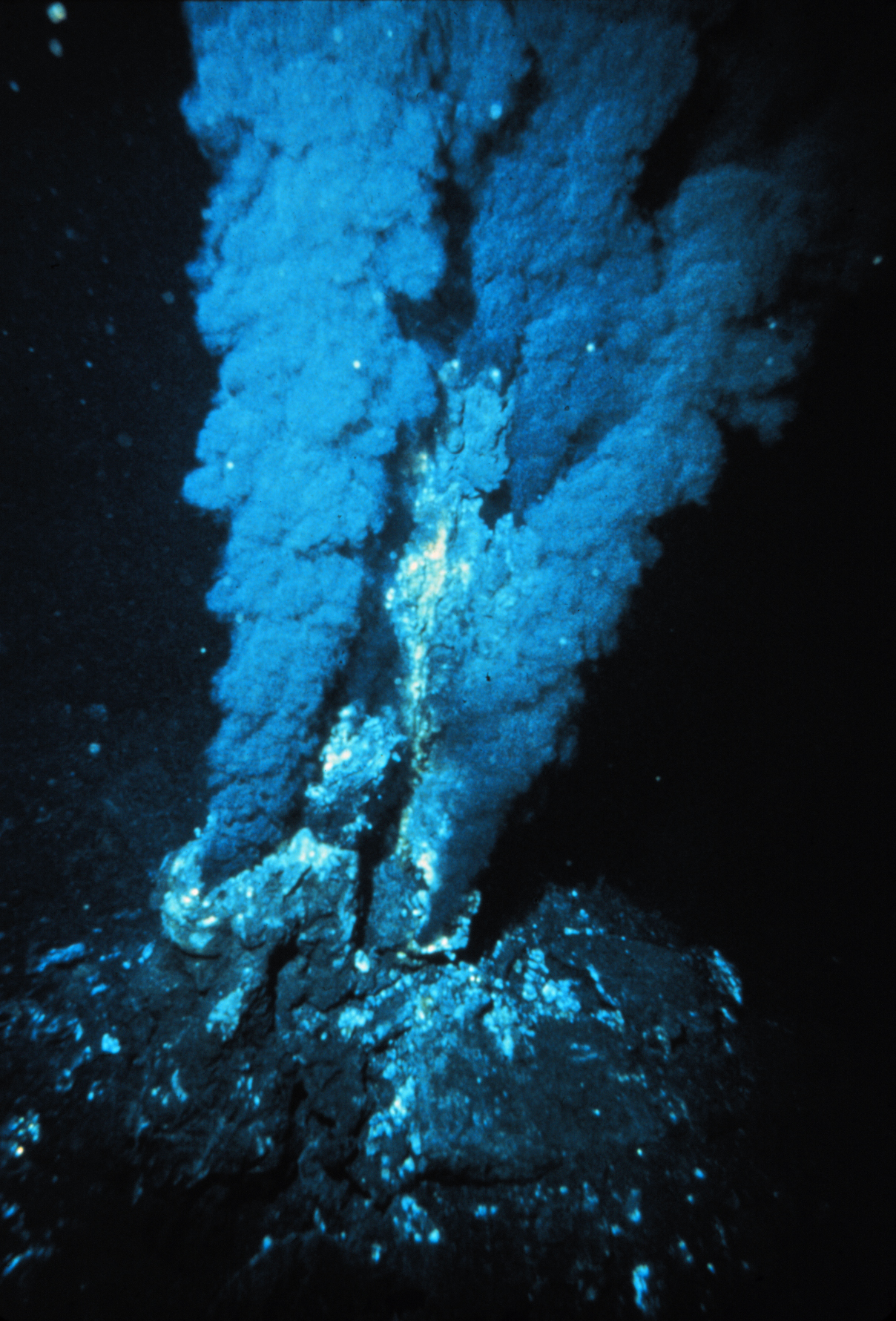
Seawater penetrates in to the newly formed ocean floor on the flanks of the ridge, and is heated at the spreading centre. This hydrothermal water dissolves elements from the mafic rock as it rises up through pore spaces in the ocean floor. As it emerges and cools it may deposit these elements in hydrothermal mineral deposits on and within the ocean floor. Hydrothermal vents are places where this hot water emerges into the oceans interaction with the cold, oxygenated water of the oceans may lead to the formation of clouds of metallic mineral particles. Some of these vents have been nicknamed black smokers from the clouds that seem to be coming out of the ocean floor. In many cases they have unusual biological communities dependent on chemosynthesis for their energy.
It turns out that rare examples of ocean floor are preserved on land, caught up in mountain belts during plate collisions. These slices of ocean floor are known as ophiolites, and allow us to view the make-up of the oceanic lithosphere[1]. Well known ophiolites occur in the Troodos Mountains of Cyprus, in the Semail Mountains of Oman, and in the Bay of Islands in western Newfoundland, Canada. Some of these contain mineral deposits, particularly volcanic massive sulphides of copper and other elements. The name Cyprus comes from the same root as copper, because much of the copper mined by the Roman Empire came from hydrothermal deposits in the Cyprus ophiolite.
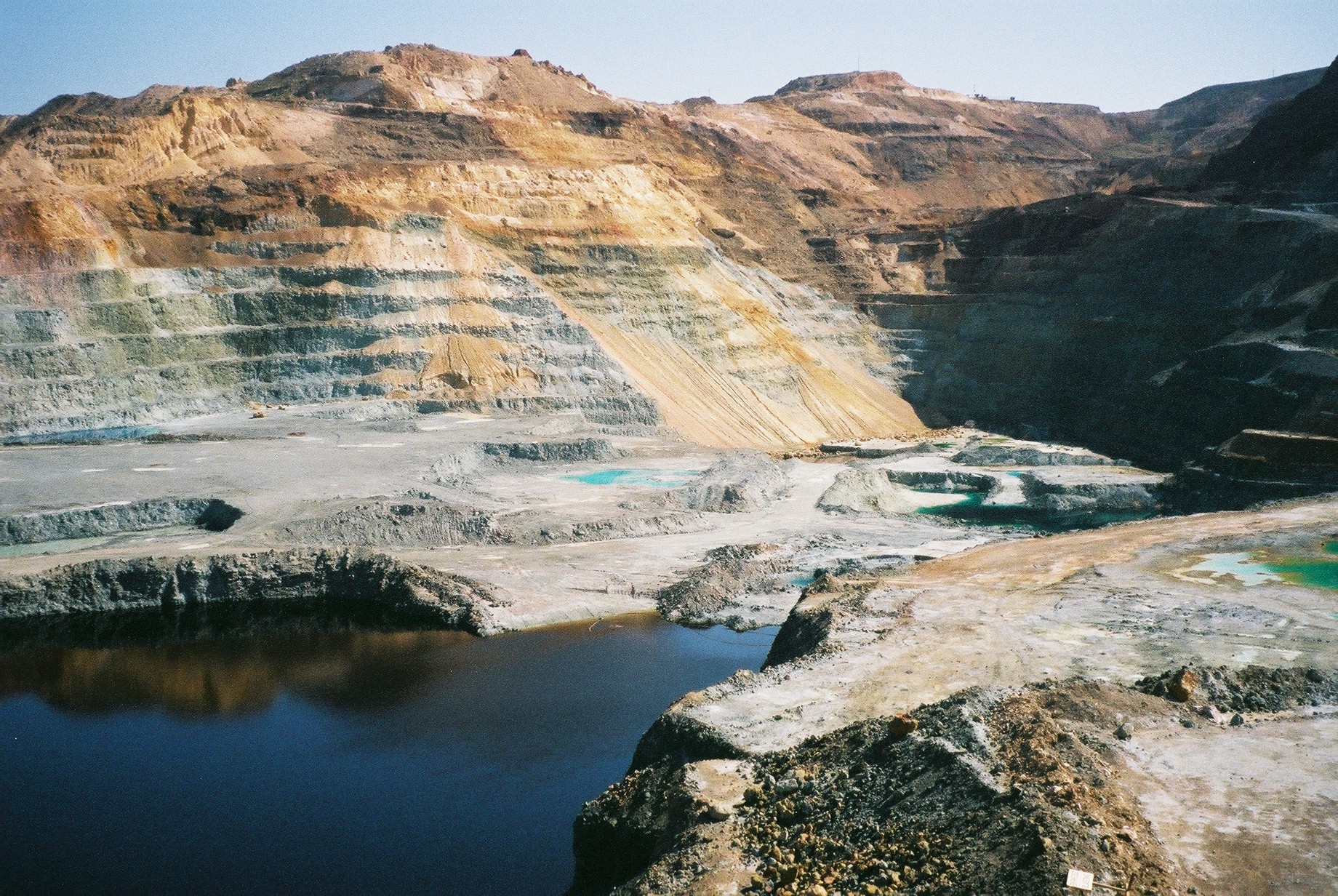
Iceland is an area where abnormally thick oceanic lithosphere (probably produced over a hotspot) is exposed above sea-level. There, hydrothermal water is used as a source of geothermal energy which makes a major contribution to the energy budget of Iceland.
Continental rifts
Most of the Earth’s present-day spreading centres originated during the Mesozoic break-up of a supercontinent by a process called rifting. Rifting is occurring in certain continents at the present-day, notably in the East African Rift, a huge valley system that cuts through Africa. An earlier example ran through Pangea along the line of the modern Atlantic. Rift valleys mark places where the crust is being slowly stretched. Eventually this stretching may lead to the development of a new ocean. This has already happened to portions of the East African Rift, producing new oceanic lithosphere, only a few million years old, in the Red Sea and the Gulf of Aden.
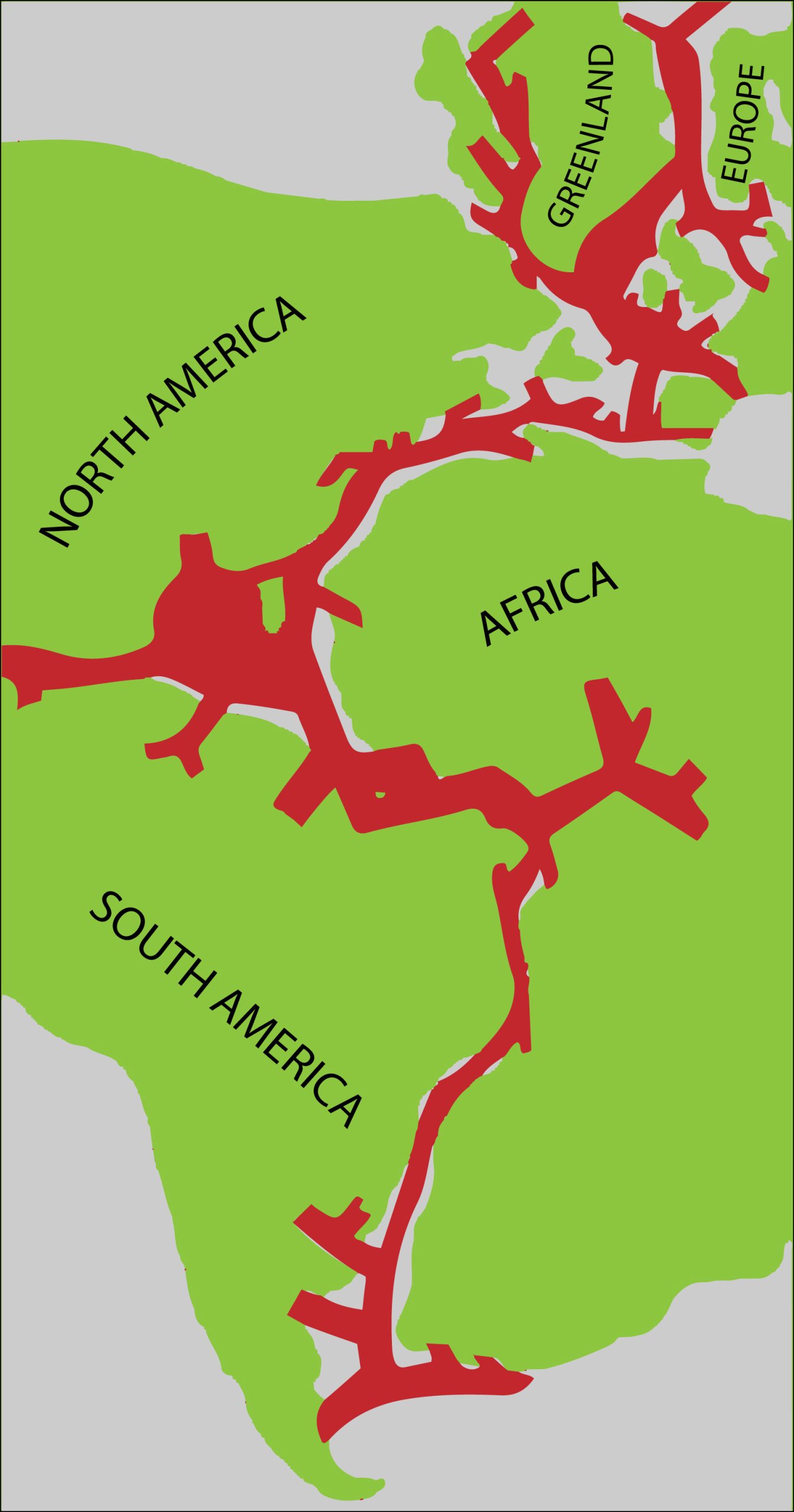
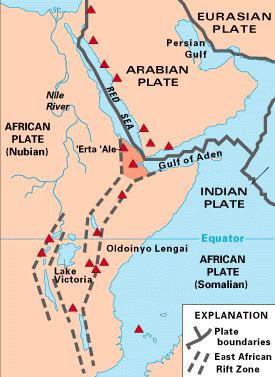
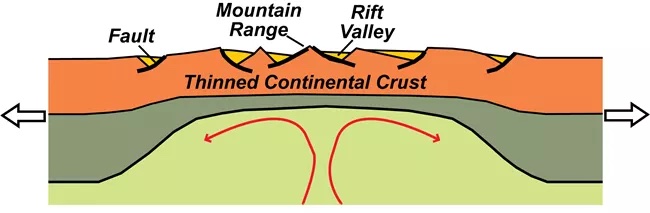
In a rift valley, the brittle upper crust is extended by faults. Because of isostasy the valley in the Geosphere surface is matched by a rise in the Moho and in the lithosphere–asthenosphere boundary. Asthenosphere rises adiabatically (without loss of heat) into the space formed as the continent rifts apart. The fall in pressure causes partial melting, although the amount of partial melting is less than at a typical mid-ocean ridge because the rise is slower. However, on its wayy up, the mafic magma may melt the continental crust, producing felsic magma. Many rifts are therefore characterized by bimodal igneous rocks: mafic and felsic rocks without many intermediate rocks.
The hot, low density rocks of the continental crust on either side of the rift sit high because of isostasy.
Passive continental margins
Eventually, the two sides of a continental rift may separate, and ocean-floor spreading may start, as has happened in the last few million years to form the Red Sea. As the ocean widens, the two sides of the rift are preserved as passive continental margins (i.e. continental margins that are not plate boundaries).

Passive margins cool over time after rifting and therefore gradually subside because of isostasy. In most passive margins, sedimentation keeps pace with subsidence producing a wide shallow sea called the continental shelf. Outboard of the continental shelf, the continental slope and continental rise overlie the buried transition between continental and oceanic crust.

Thick accumulations of marine fine-grained sediment on passive continental margins may trap organic matter. The action of geothermal heat on that organic matter may convert it to oil or natural gas. Because oil and natural gas are less dense than water (which fills most porosity in the subsurface) they slowly migrate upwards through permeable and porous units until they either escape (as seeps) from the geosphere or become trapped by impereable units.
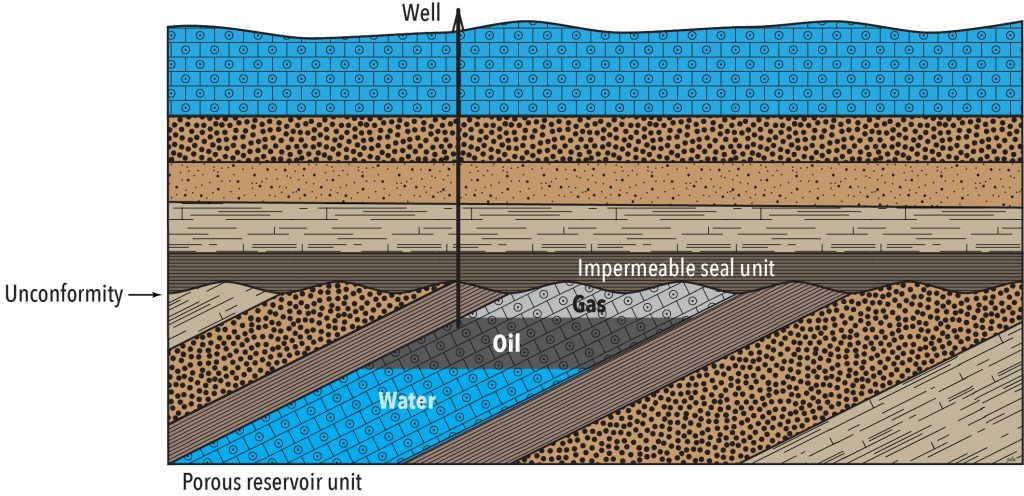
Oil and gas may accumulate in porous units of sandstone or limestone. The hydrocarbons flow out to the surface if a well is drilled into them. These are known as conventional hydrocarbon resources. Hydrocarbons trapped in impermeable mudstone or shale do not flow out unaided; horizontal drilling and hydraulic fracturing are typically used to increase the permeability of such rocks in order to extract hydrocarbons. These techniques have been developed more recently and these hydrocarbons are known as unconventional oil and gas resources.
Where rivers empty onto continental shelves, thick piles of clastic sediment called deltas may form. In humid environments, deltas and other low-lying wetlands may be support by forests, which produce deposits of peat that become converted to layers (known as seams) of coal once buried, through compaction and the action of geothermal heat.
Humans use coal, oil and natural gas — fossil fuels — as energy sources, producing CO2 and H2O when they are oxidized.
Carbon dioxide impact, per gigajoule of energy, depends on proportion of carbon to hydrogen in the fuel. Coal is mostly carbon, so produces the most CO2 per gigajoule of energy. Oil contains more hydrogen relative to carbon, so its CO2 impact is somewhat less, while natural gas, mostly methane (CH4) produces the least CO2 per gigajoule amongst fossil fuels.
In addition to fossil fuels, passive continental margins have provided many other resources to humans, particularly those in coastal communities. For example, limestone and mudstone are abundant in many successions of strata from passive continental margins. These two rock-types are reacted together to make cement and concrete (concrete is cement mixed with coarser clastic sediment like sand or gravel). After the burning of hydrocarbons, cement manufacture is the second largest anthropogenic source of CO2 accounting for around 8% of anthropogenic CO2 release into the atmosphere.
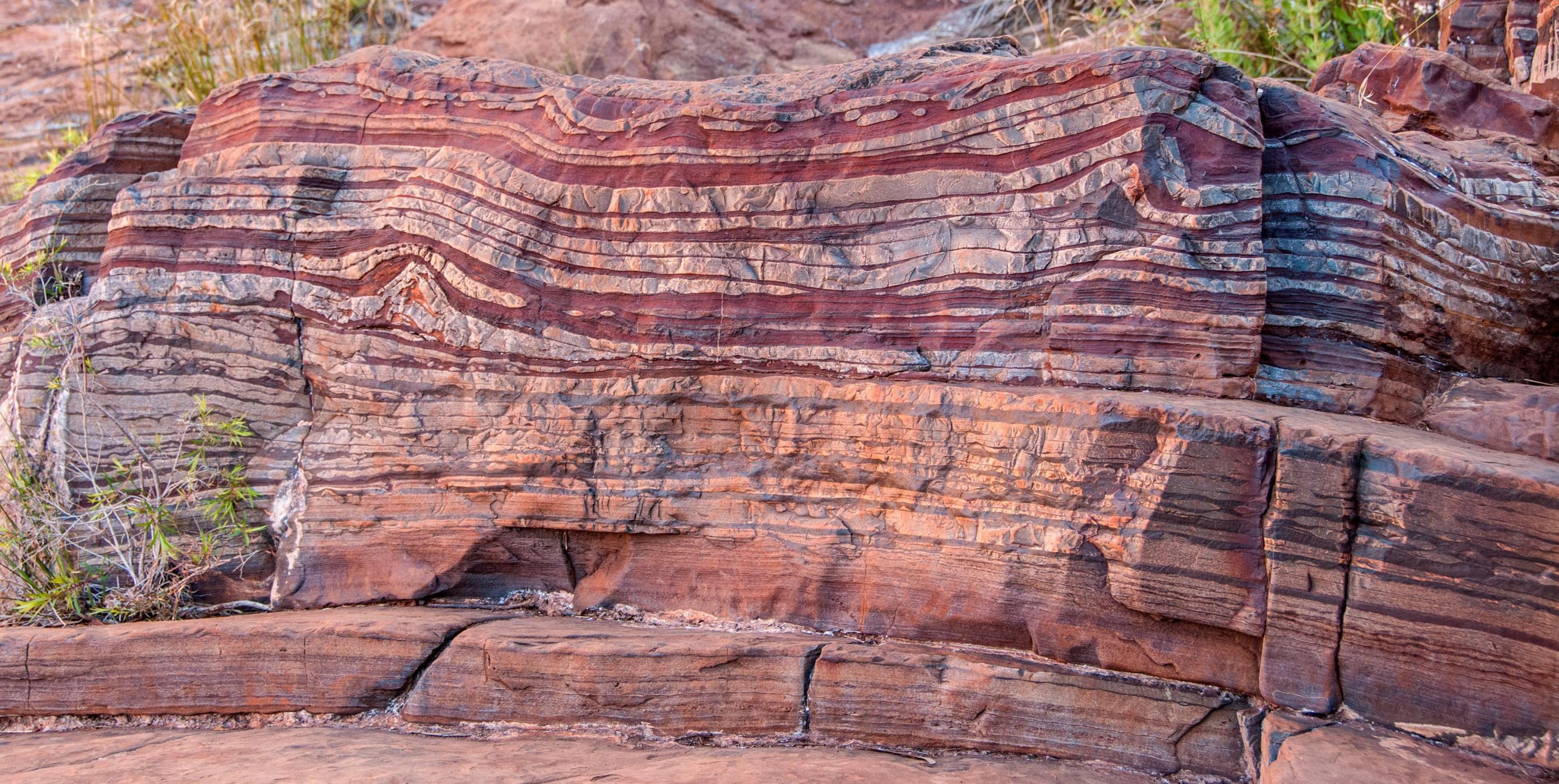
Farther back in the record of Earth history, Proterozoic passive-margin sediments include thick units of banded iron formation, the largest sources of iron used in human industry.
Transform faults: plates moving past each other
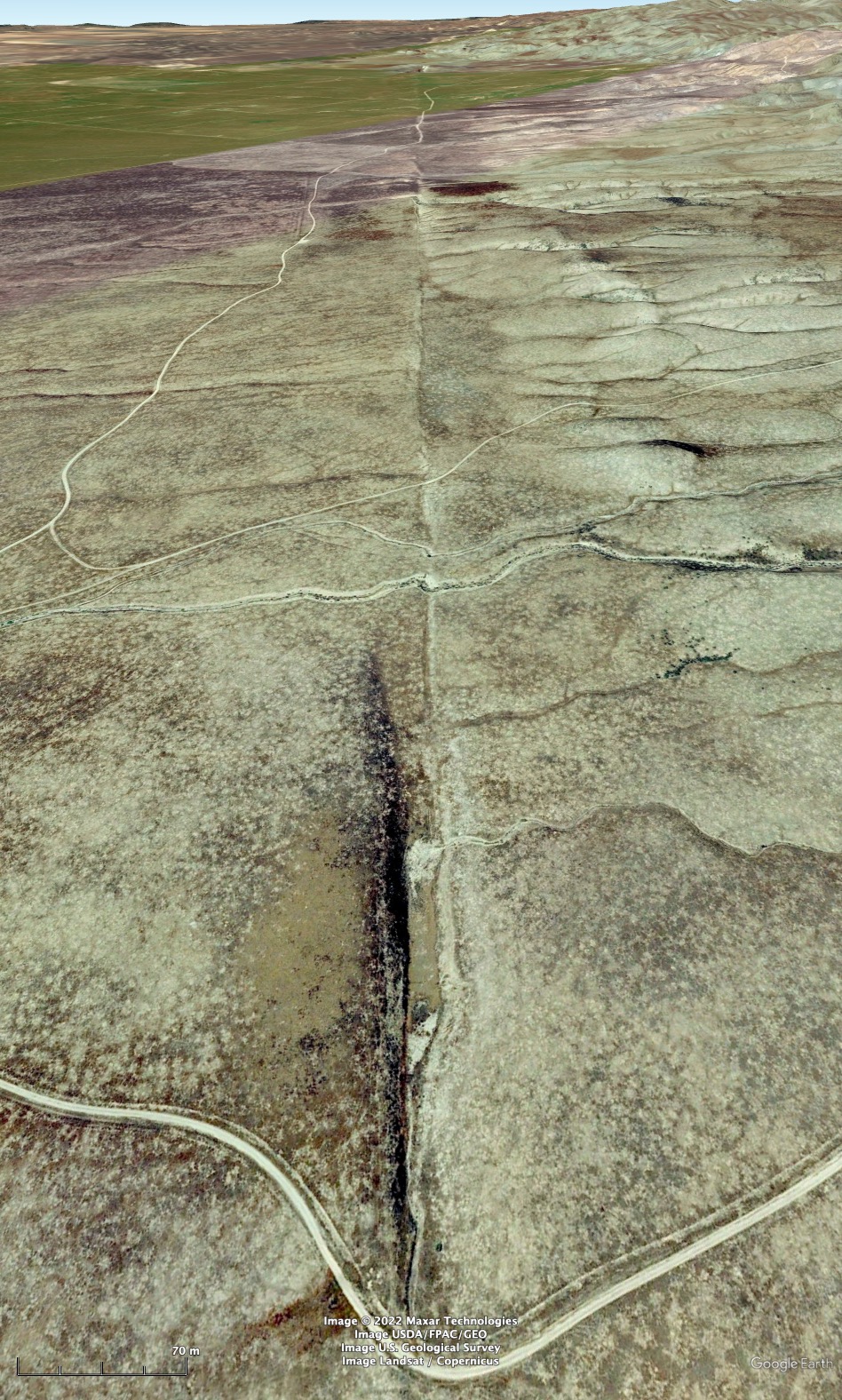
Transform faults are plate boundaries at which the relative movement between two plates is parallel to the boundary. Transform faults occur in both continental and oceanic lithosphere.
The San Andreas Fault, the best-known example of a transform fault, is dextral. The Arava–Jordan fault system that passes through the Dead Sea, is an example of a sinistral transform fault.
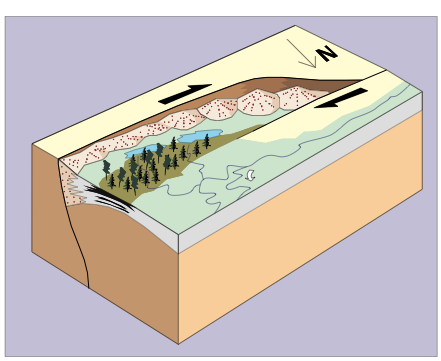
Many transform faults are remarkably straight. Typically, the zone of deformed rocks along a transform fault is very narrow. However, small bends can occur on transform faults, and depending on their orientation, they may be restraining bends where the two sides of the plate push together slightly, or releasing bends, where the plates pull apart. In this second case, a small, rapidly subsiding pull-apart basin may form. Pull apart basins tend to trap sediment, and they may contain hydrocarbon deposits. The Los Angeles Basin in California is an example of a sedimentary basin formed in association with a transform fault. It contains substantial amounts of oil and natural gas. In Nova Scotia, Canada, the Stellarton Basin formed at a dextral transform fault that existed in the Paleozoic Era. Because Nova Scotia was in the tropics aat the time, tropical rain forest were able to deposit numerous peat layers, which became coal seams that were intensively mined in the 20th century, continuing to the present day at the time of writing (2024).
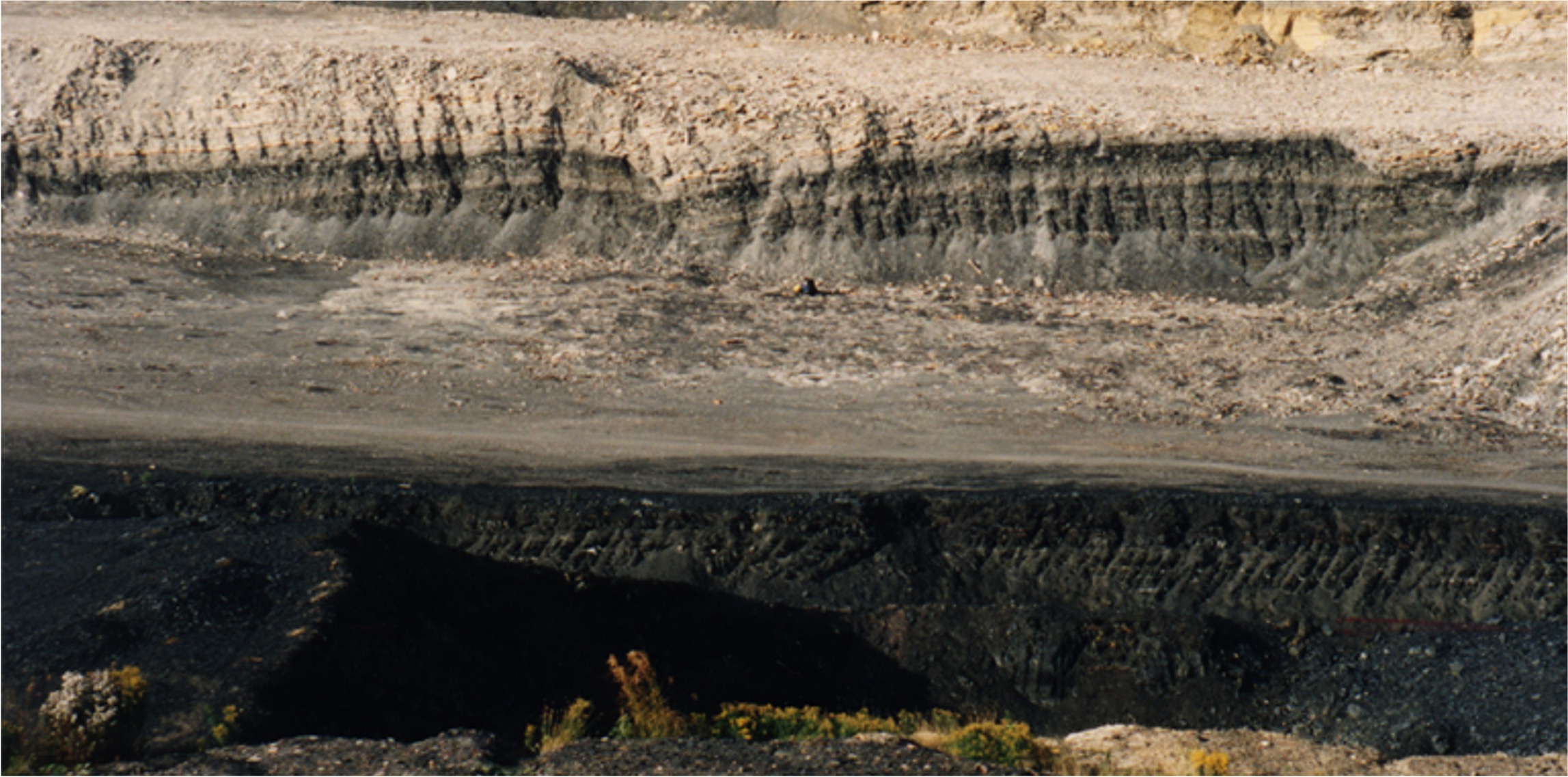
Convergent tectonic environments: plates moving towards each other
Convergent tectonic environments are those where plates are converging. These are some of the most varied and dynamic environments, producing earthquakes, volcanoes, and a variety of resources that are mined by humans. Convergent environments are responsible for the highest mountains and the deepest ocean trenches on Earth. There are a number of types of convergent tectonic environments, depending on the presence of oceanic or continental crust on either the lower or the upper plate of the convergent system. We here examine four different permutations of continental and oceanic crust in convergent environments. At the end of this section, we will look at foreland basins, depressed areas of the lithosphere where a plate is held down by the isostatic effect of adjacent mountains.
Subduction zones and volcanic arcs
Subduction zones are sites where plates continuously converge. The subducted plate in most subduction zones carries oceanic crust, returning it through the subduction process back into the mantle.
Subudction zones produce the largest earthquakes, accounting for all the magnitude 9 (or higher) earthquakes recorded since the advent of modern seismology in the mid-20th century.
What happens to the subducted lower plate?
The rocks of the downgoing plate are subjected to metamorphism at high pressure, but relatively low temperature, because it takes millions of years for the thick slab of oceanic lithosphere to warm up to the temperature of the surrounding mantle.
Under such conditions, feldspar, usually one of the most abundant components of igneous rocks, becomes unstable. If there is water in the rocks of the downgoing slab, ferromagnesian minerals may absorb sodium from feldspar to produce a distinctive blue chain silicate mineral glaucophane. Such metamorphic rocks are known as blueschists. A second very distinctive metamorphic rock type occurs where high pressure conditions affect mafic rocks without water. Aluminum from feldspar combines with other silicates to form the red mineral garnet, while sodium dissolves in green pyroxene. The resulting red and green metamorphic rock is called eclogite.This mineral assemblage is only stable below about 45 kilometres depth, where the pressure is 1.2 GPa or higher.
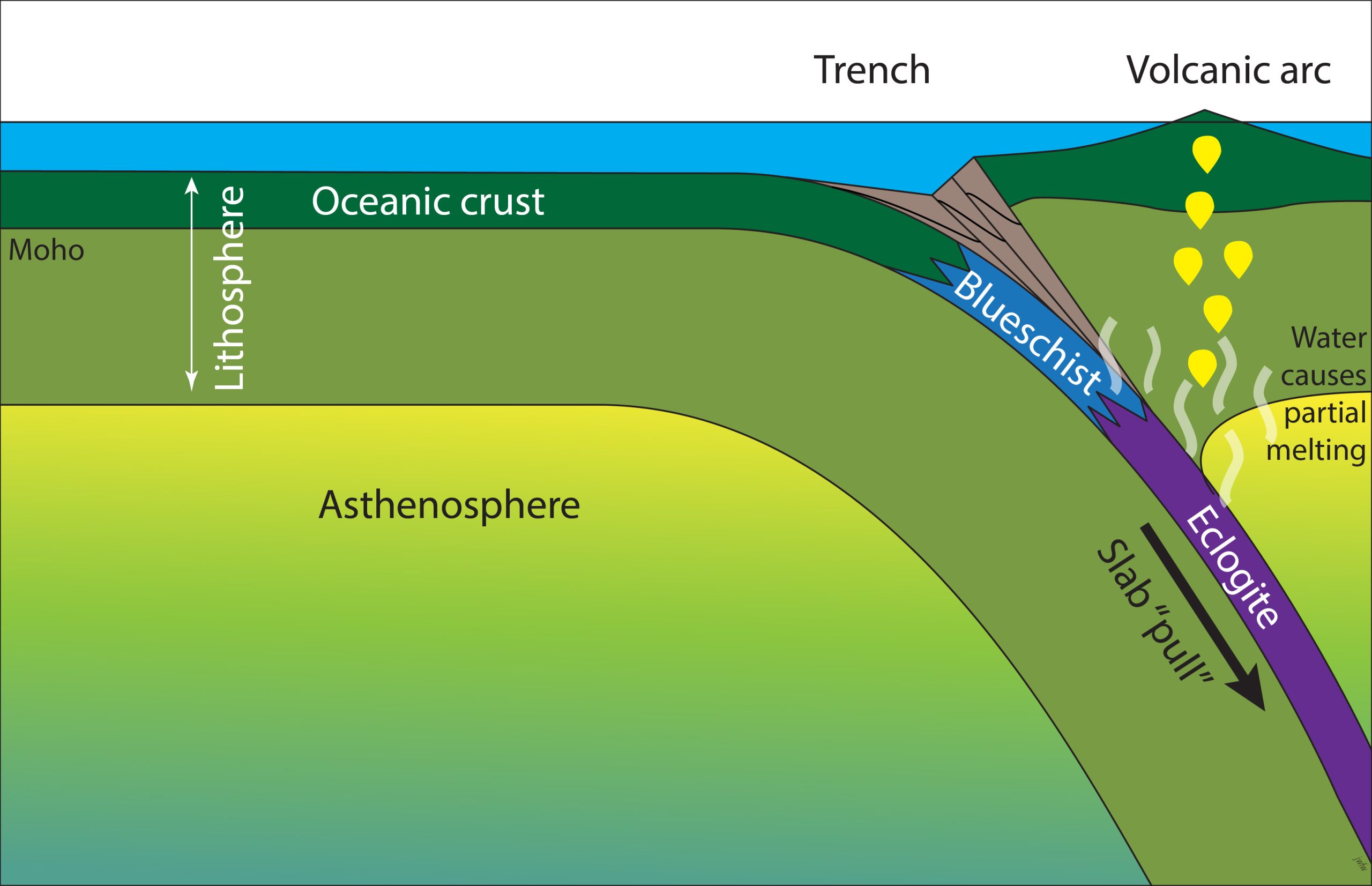
Although both these metamorphic rock types have the same overall composition as mafic igneous rock like basalt and gabbro, they are much denser. They are even denser than typical mantle peridotite. It is therefore believed that the formation of eclogite plays a role in driving plate tectonics, because it makes the slabs of ocean floor that hang down below subduction zones are denser than the surrounding mantle, and therefore help to pull the subducted plate down..
Blueschist and eclogite may be brought back up to the surface under exceptional circumstances, particularly if subduction stops as a result of plate rearrangment. They are quite rare rocks but, when found, are clear indicators of ancient subduction zones.
Volcanic arcs and active continental margins
Initially, the rocks of the downgoing plate typically contain quite large amounts of water, locked up in hydrous minerals formed on the ocean floor. As they are metamorphosed into eclogite, these rocks lose water, which flows into the overlying mantle. As explained in the section on igneous rocks the addition of water to mantle peridotite causes partial melting, forming magma that rises to the surface and is responsible for the formation of a volcanic arc on the upper plate of the subduction zone. As you might expect, those volcanoes are rather different from those formed at mid-ocean ridges, because the magmas in subduction zones contain significant amounts of water together with traces of material derived from the downgoing slab. That causes them to evolve somewhat differently as they rise towards the surface. Typically they form intermediate magma, and the water they contain makes them more explosive than mid-ocean ridge volcanoes. Typical subduction-zone volcanoes are conical stratovolcanoes like Mount Fuji in Japan or Mount Rainier in Washington State, USA. Two examples in Indonesia, Krakatau and Tambora, were responsible for huge eruptions in the 19th century CE that put so much tephra into the atmosphere that they significantly cooled Earth’s climate for several years. A similarly powerful eruption of Mount Pinatubo in the Philippines in 1991 also had a detectable cooling effect on the climate.
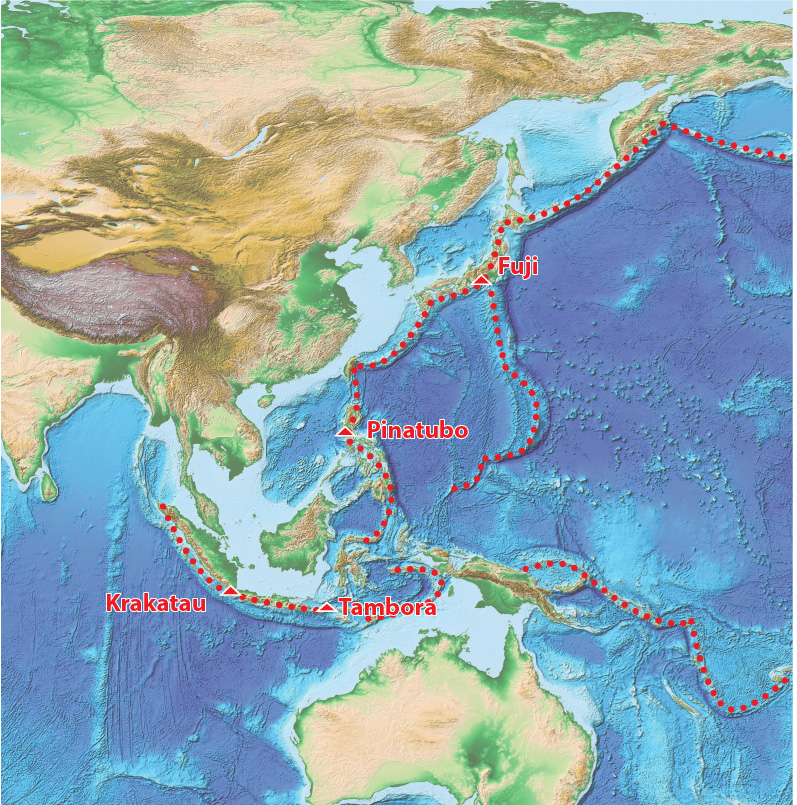
The overlying plate, above the subduction zone, experiences slightly different phenomena depending on whether it is continental or oceanic.
Oceanic Island Arcs
In the situation where the overlying plate is oceanic, it typically carries a curved chain of volcanoes known as an island arc. There are many examples around the West Pacific. Island arc volcanoes erupt mafic magma (formed by partial melting of peridotite), but they also erupt intermediate magma. The intermediate compositions probably result from the long journey that these magmas have taken (typically about 100 km) from their source in the mantle wedge. As the magma it rises towards the surface under the influence of water, some crystals of ferromagnesian minerals settle out, leaving the magma richer in silica, giving it a more intermediate composition. This process of magma differentiation is probably very important in the overall evolution of the Earth’s crust. Most geologists who have worked on the overall evolution of the Earth think that island-arc formation this is one of the main process is whereby the Earth’s silica-rich continental crust has formed over time.
Continental volcanic arcs and active margins
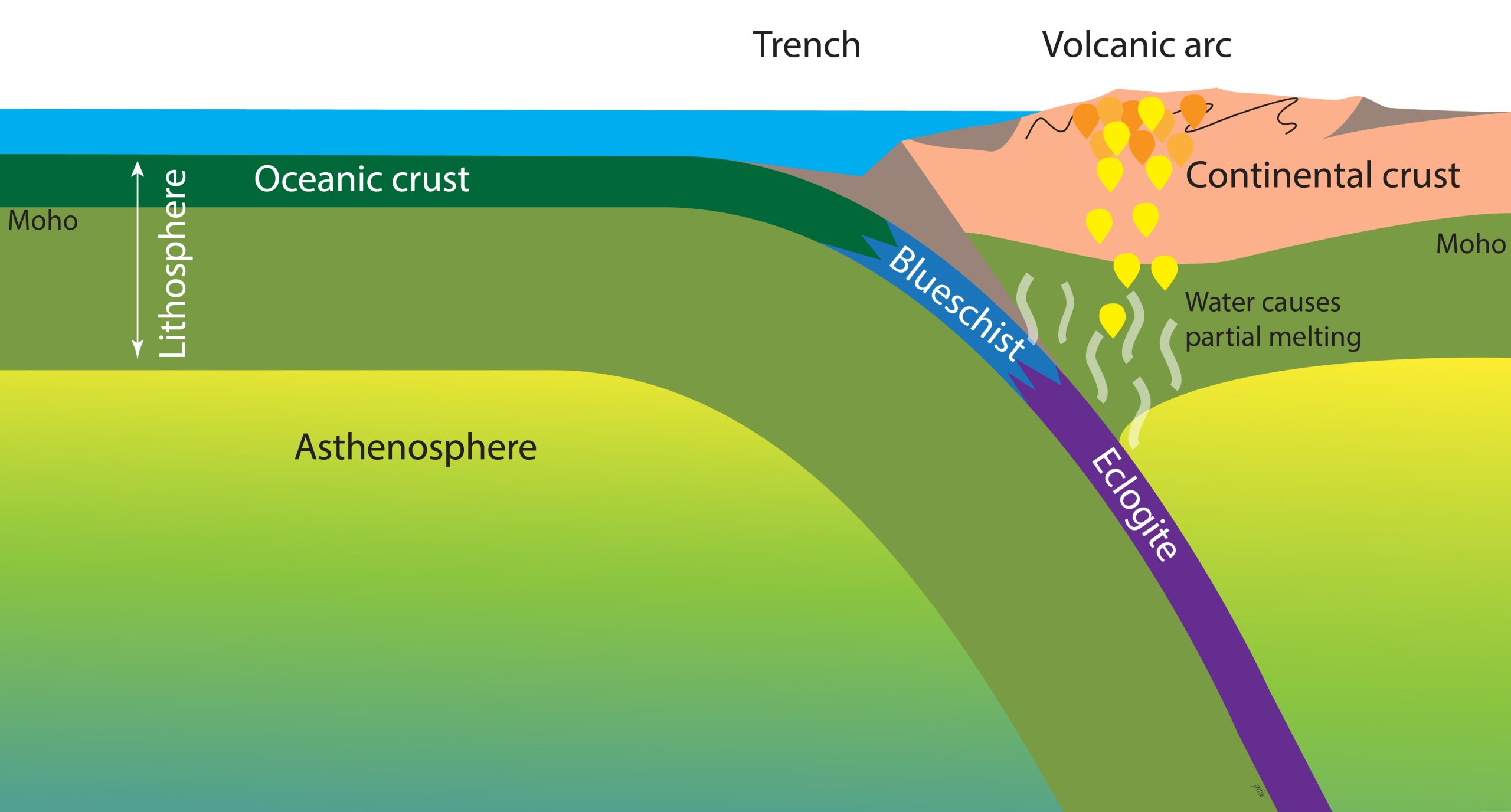
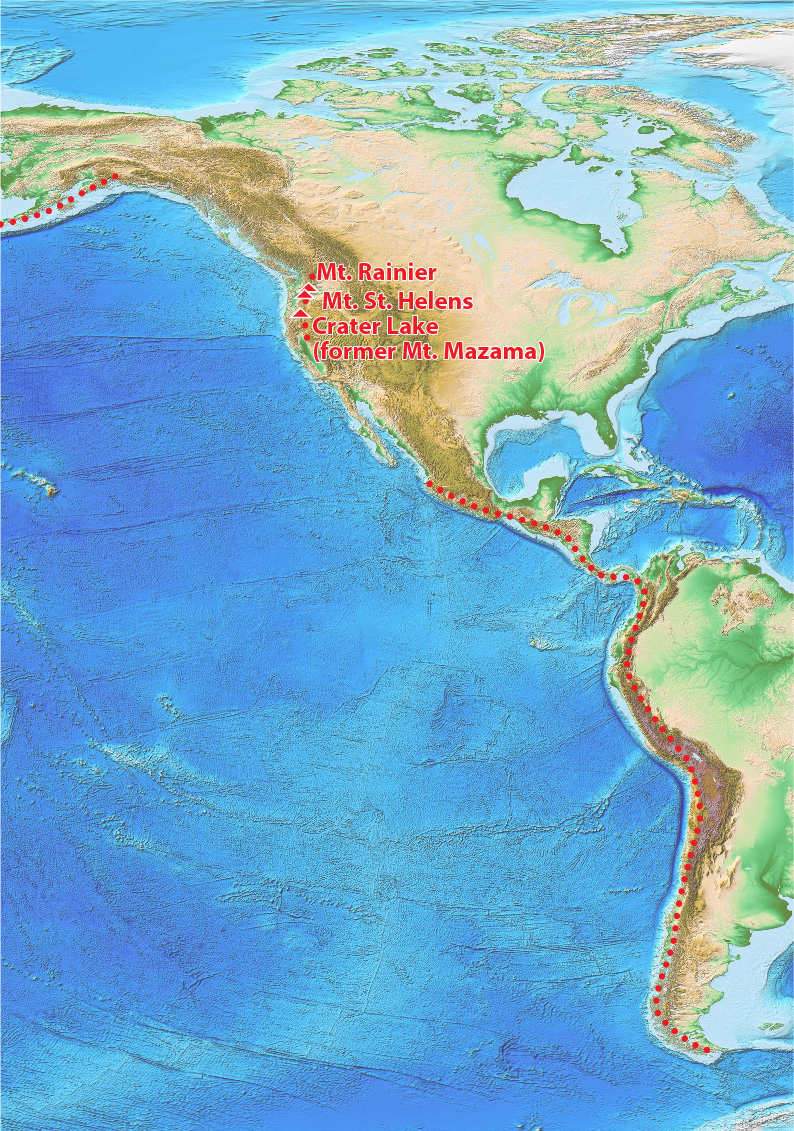
Where the upper plate in a subduction zone is continental, as occurs at the western edge of South America and parts of North America, volcanoes tend to be even more silica rich, resulting in intermediate to felsic compositions. This results both because of the long journey that the magma takes to the surface (allowing plenty of time for differentiation) and also because the magma may melt parts of the silica-rich continental crust that it passes through. Intermediate to felsic volcanic activity has produced the famous volcanoes of the Cascade Range in western North America, including Mt. St. Helen’s, Mt. Rainier, and the former Mt. Mazama in Oregon, which exploded about 8000 years ago forming Crater Lake, and covering the Pacific northwest with tephra, some of which is preserved as far north as Edmonton, Alberta.
The western margins of both the North and South American continents are also plate boundaries. These continental margins are therefore known as active continental margins. In addition to the volcanic activity at the surface, a lot goes on beneath the surface in these areas. As you might expect, many of the volcanoes sit above bodies of magma that cool down before reaching the surface to produce igneous intrusions. The magma typically contains a lot of water, and hot water penetrates into the surrounding rocks, carrying with it dissolved metals, which may be deposited within the pore spaces to produce mineral deposits. One very important category of deposit is called porphyry copper. Porphyry is a name for an intrusive igneous rock that formed only a short distance below the surface; it contains a mix of coarse crystals (recording slow cooling of the magma while it was deep down) and finer grains formed by more rapid cooling of the remaining magma near the surface. Copper is present in these deposits mainly as sulphides. Some porphyry deposits also contain silver, gold, and molybdenum. One of the challenges in extracting copper from these rocks (as is also true of volcanic massive sulphide deposits in oceanic crust) is that the sulphur has to be removed by reacting the ore with oxygen, which generates the acidic gas sulphur dioxide, a major contributor to acid rain.
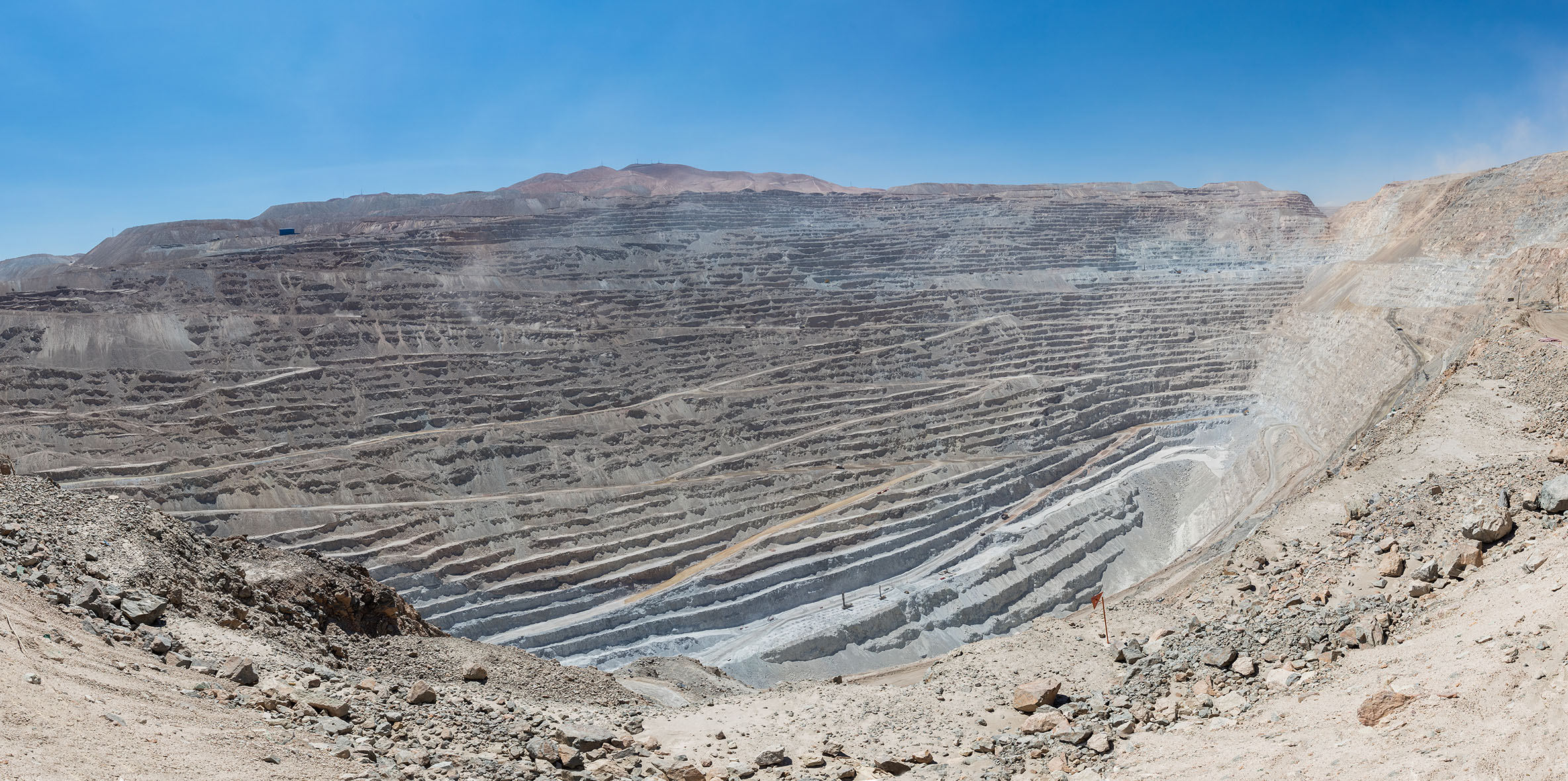
It’s clear from the map of North and South America that active continental margins are typically mountain belts. These mountains are produced by shortening of the continental crust in response to convergent plate movement. When we look at rocks that have been exposed by erosion in active continental margins, it’s common to see metamorphic rocks with fabrics, and sedimentary strata that have been shortened by folds and faults. Faults produced by shortening are typically thrust faults, sloping faults where the upper block of rock (the hanging wall) has moved up and over the lower block (the footwall). Typically this means that they place older strata on top of younger, in violation of the principle of superposition.
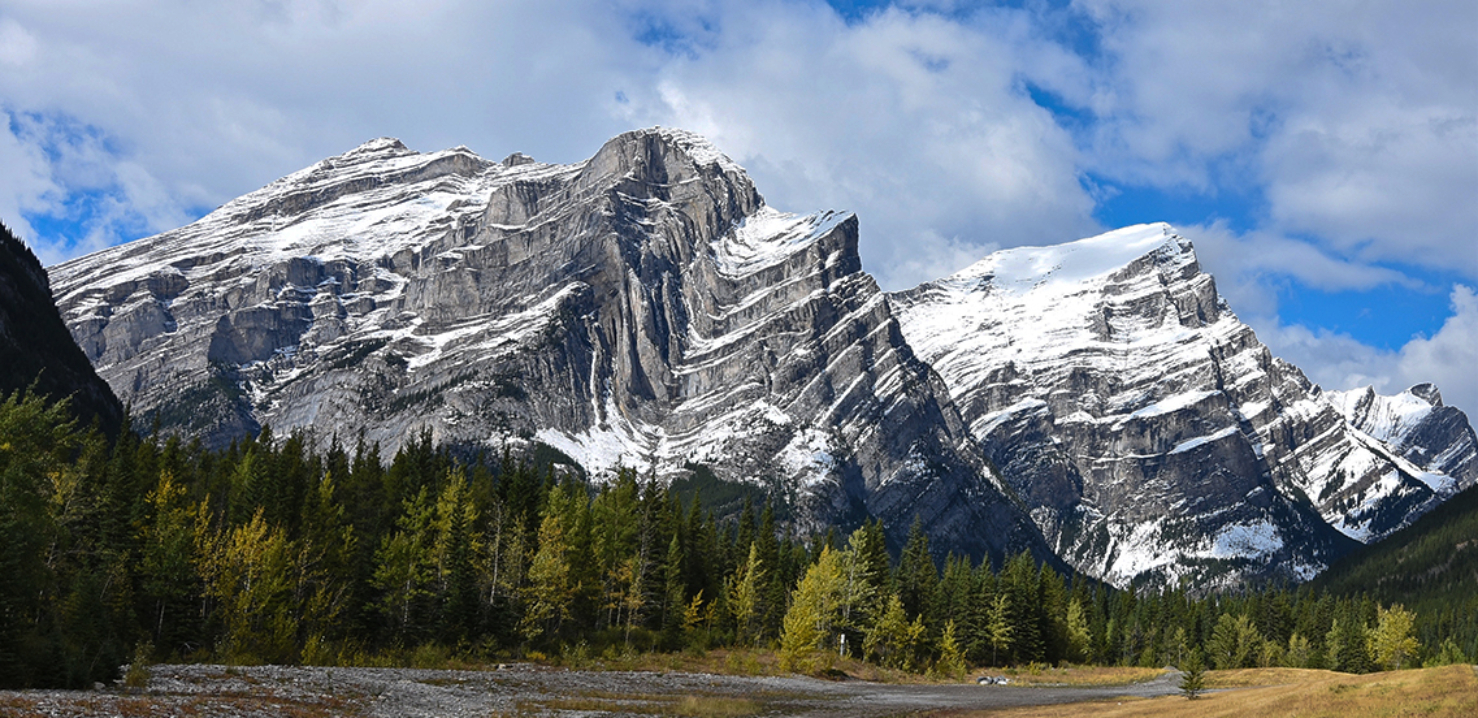
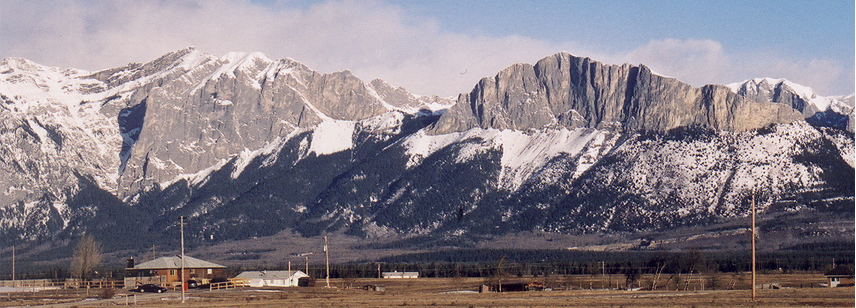
The formation of mountains by shortening of the crust is known as orogenesis, from Greek words meaning mountain formation, and the belt of mountains formed by this process is known as an orogen. In North Americal the orogen along the Pacific continental margin is known as the Cordillera or Cordilleran orogen. In South America the corresponding mountain belt along the active continental margin is called the Andes. Orogens formed like the Andes, as a result of continuous subduction of oceanic lithosphere, often for tens of millions of years, beneath an active continental margin, are sometimes called Cordilleran or Andean orogens.
Collisional orogens
In the previous sections we have distinguished two different types of subduction zone based on whether there is continental or oceanic lithosphere in the upper plate of the system, when the lower plate is oceanic. What happens if the lower plate in a subduction zone contains a continent? Because continental crust is much less dense than oceanic crust, it’s buoyant and resists being subducted because of isostasy. The downgoing continental crust may jam up the subduction zone, causing a range of mountains, a collisional orogen, to grow over a few million years. Once again, we can distinguish two possibilities based on the nature of the upper plate. If the upper plate carries an oceanic island arc, then the process is described as continent-arc collision. If on the other hand the upper plate is continental, then the process is called continent-continent collision.
Arc-continent collision
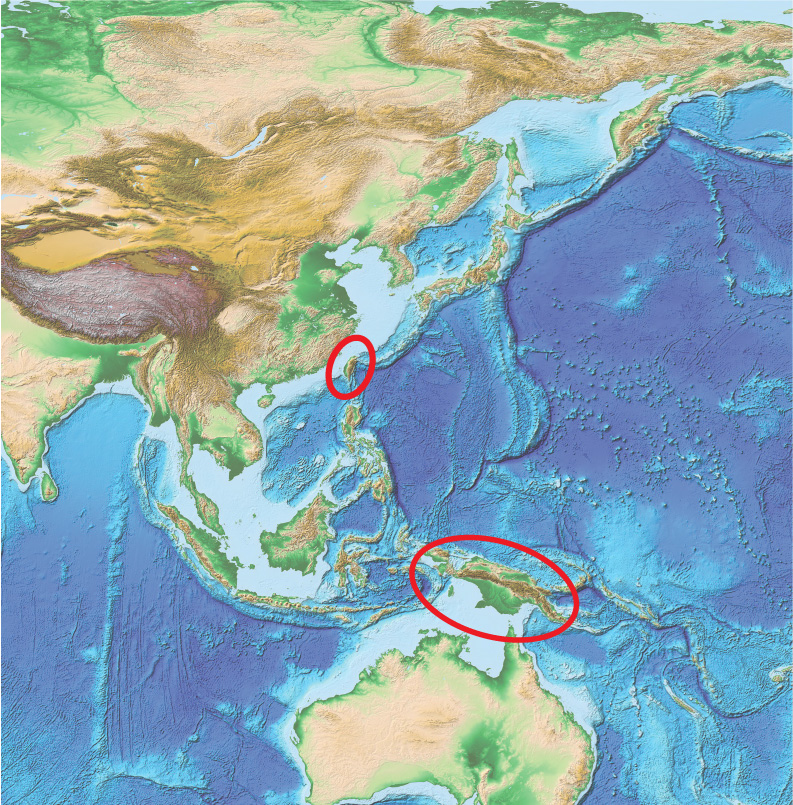
Arc-continent collision is taking place in at least two places in the western Pacific Ocean region at the present day. In Taiwan, a former island arc is colliding with the passive continental margin of China, building a new orogen on the previously passive continental margin. The area is an active earthquake belt, as thrust faults are shortening the crust of Taiwan. In Papua New Guinea, the northern passive continental margin of Australia started colliding with an oceanic island arc in the late Cenozoic Era at about 12 Ma. A complex series of collisions has thrust a large ophiolite, a slab of oceanic lithosphere, onto the continental margin. As the plates continued to converge, the buoyant Australian continental margin could not be subducted because of isostasy, so new subduction has started to pull the Pacific Ocean floor under the Australian to the south, converting it into an active continental margin.
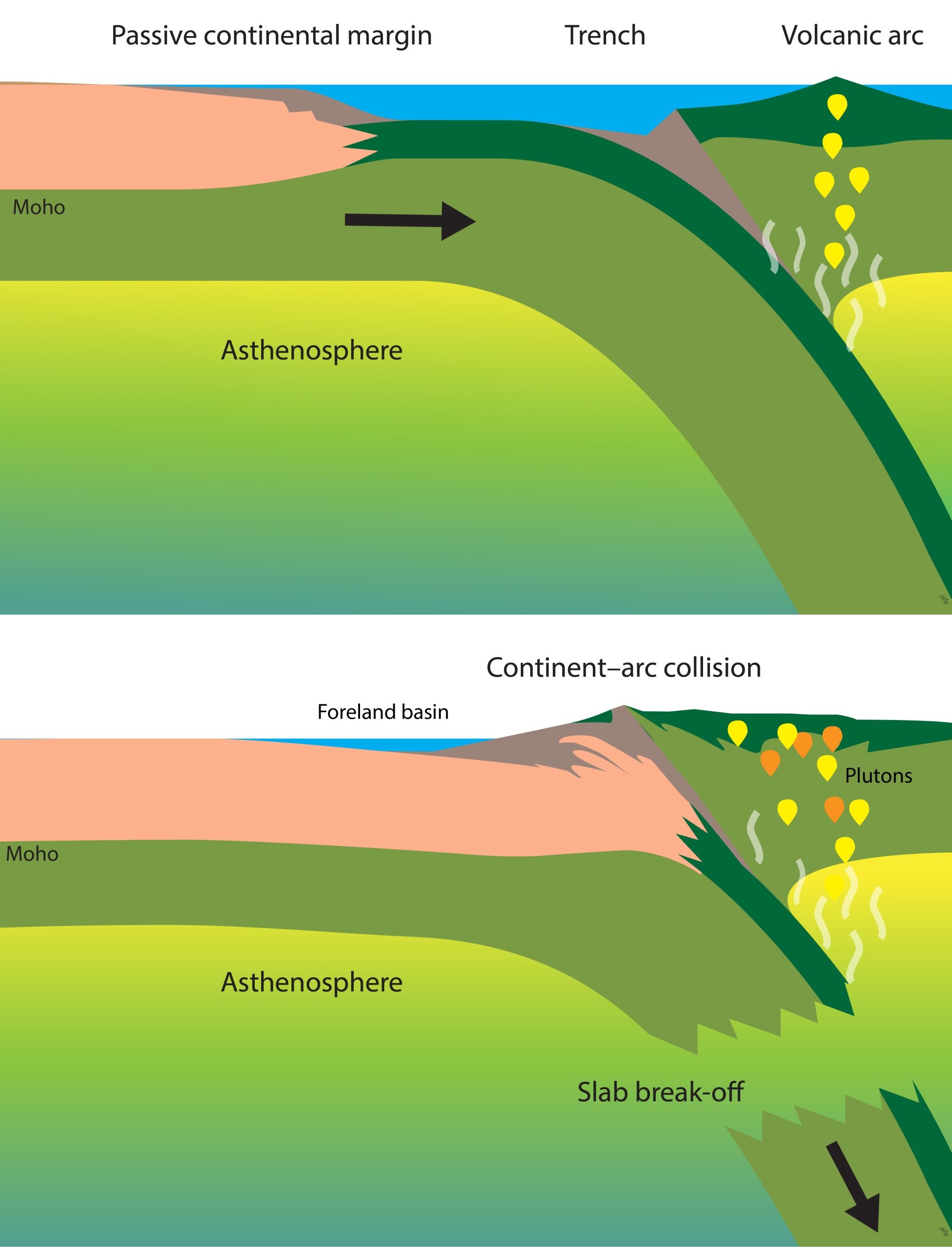
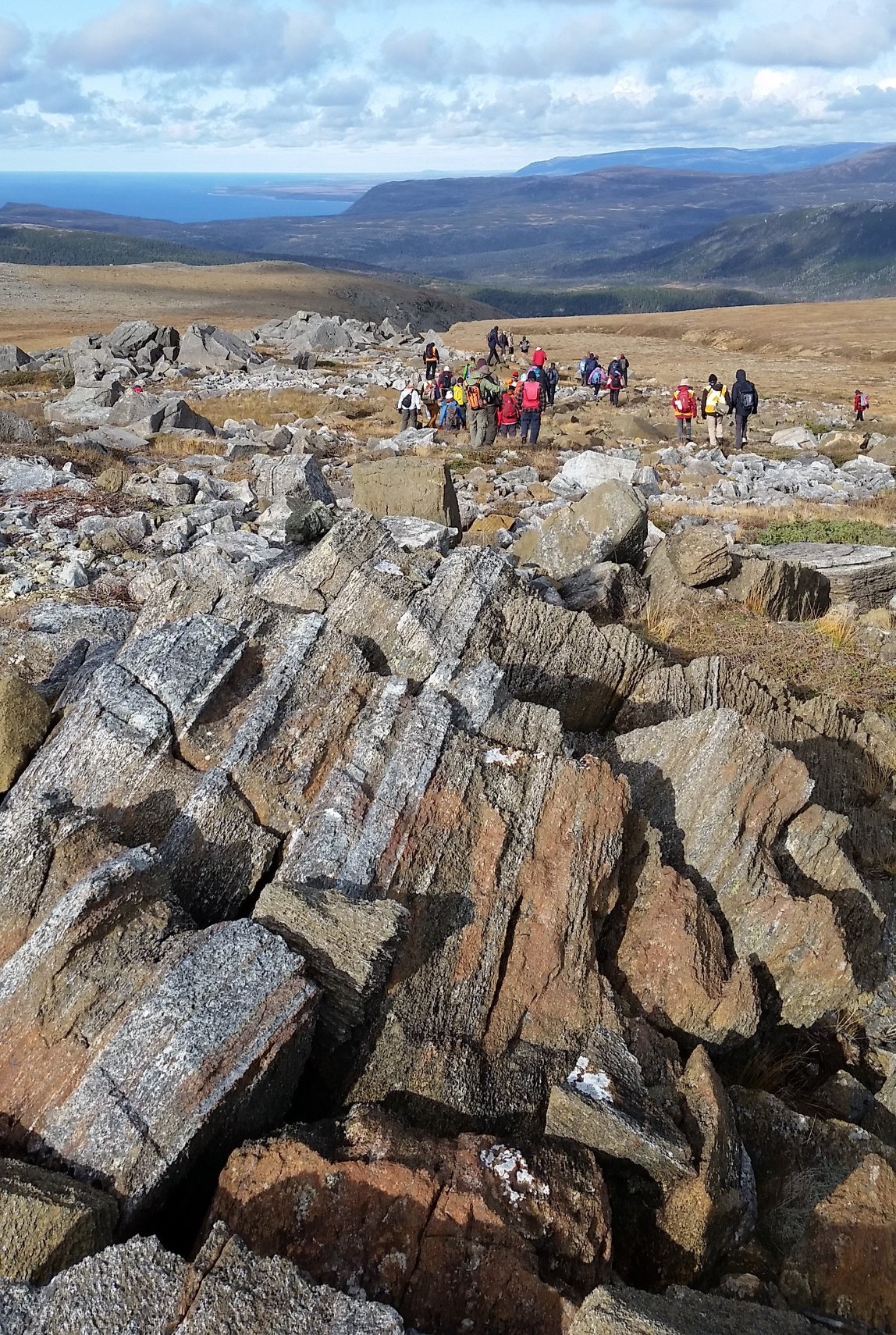
Closely similar events occurred along the eastern margin of North America, but about 470 million years ago in the early Paleozoic Era. In western Newfoundland, a slab of oceanic lithosphere forms the Bay of Islands ophiolite, which was thrust onto the margin of ancient North America during an continent-arc collision.
Ophiolites in orogens preserve the same types of mineral deposits as are found in the ocean basins, particularly including volcanic massive sulphide deposits. (See the section on spreading centres for more information.)
Continent-continent collision
Continent-continent collision occurs when continental lithosphere arrives at both sides of a subduction zone. Continent-continent collision has been responsible for the largest orogen on the modern Earth, the Himalaya, together with the Tibetan Plateau which lies to its north. Collision is believed to have doubled the thickness of the continental crust beneath the Himalaya and the Tibetan Plateau, beneath which the Moho occurs at about 70 km depth. As in other types of orogen, this thickening is achieved in part by the motion of thrust faults, and movement on these faults leads to damaging earthquakes.
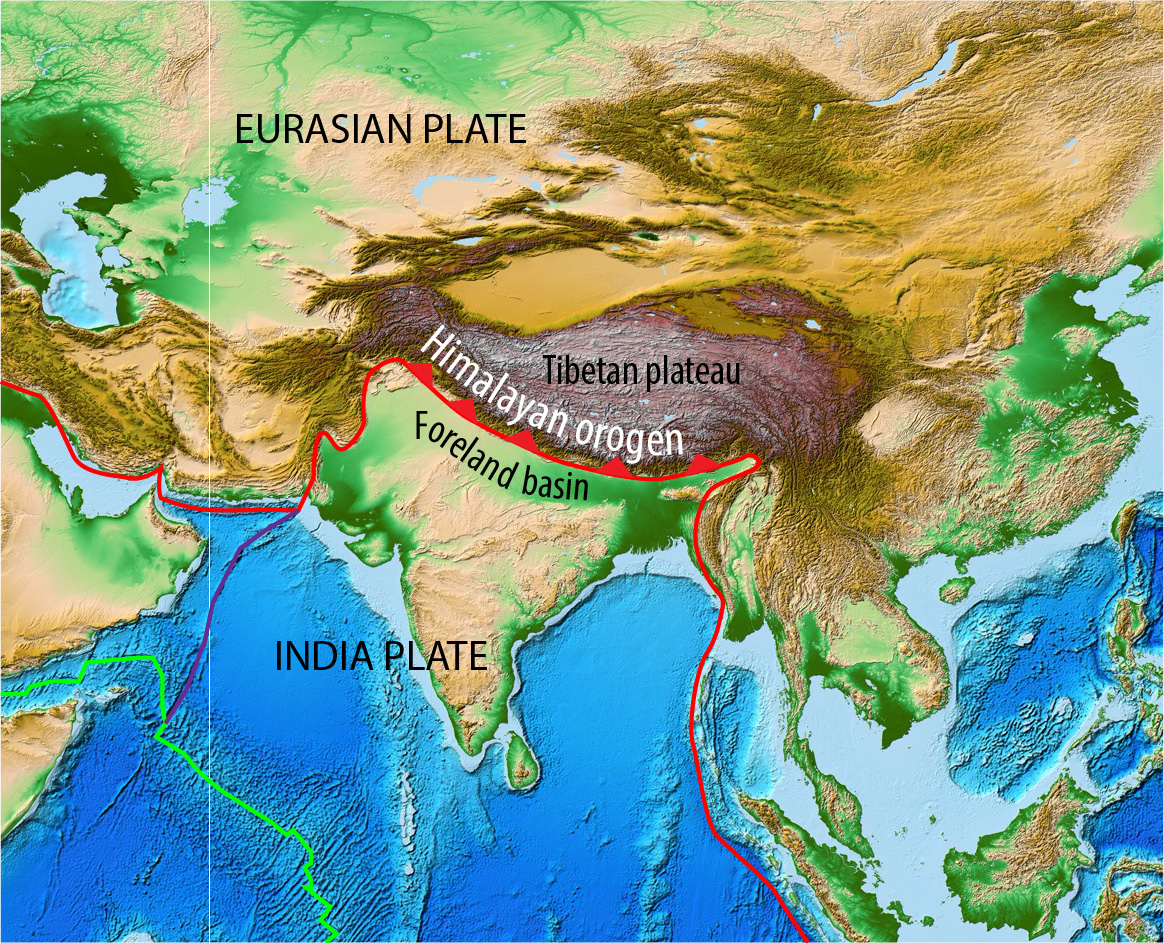
Thickening of continental crust, especially sedimentary rocks containing water, can lead to partial melting, as wet rocks are subjected to increasing temperatures and pressures. The resulting magma is felsic, and produces large plutons of granite. Additional melting can happen when the lithosphere slab breaks off and hot asthenosphere rises into the space beneath the continental crust.
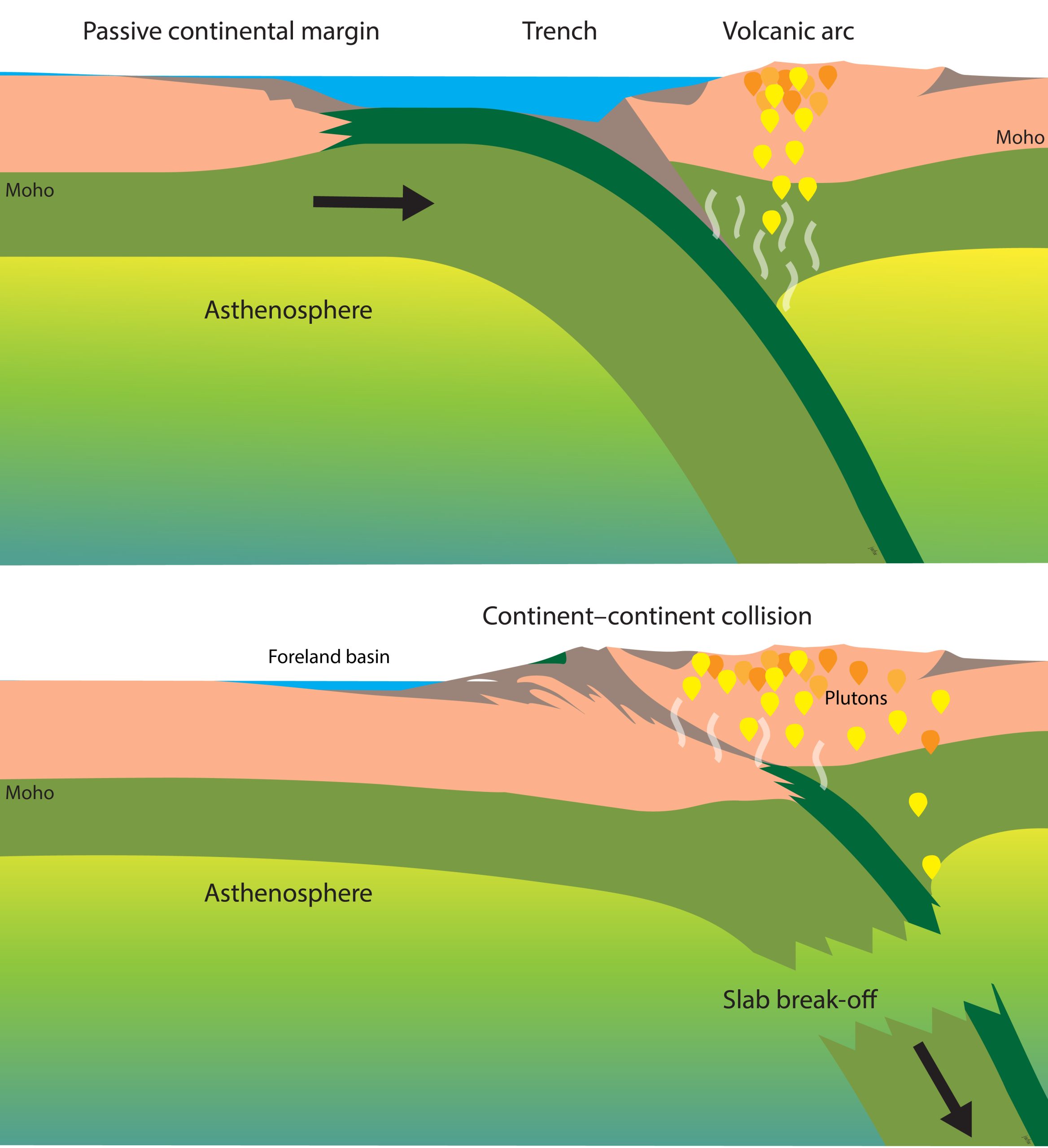
Felsic intrusive rocks in collision zones are sometimes are mined for rare metals including lithium, a metal of increasing strategic importance for the production of batteries.
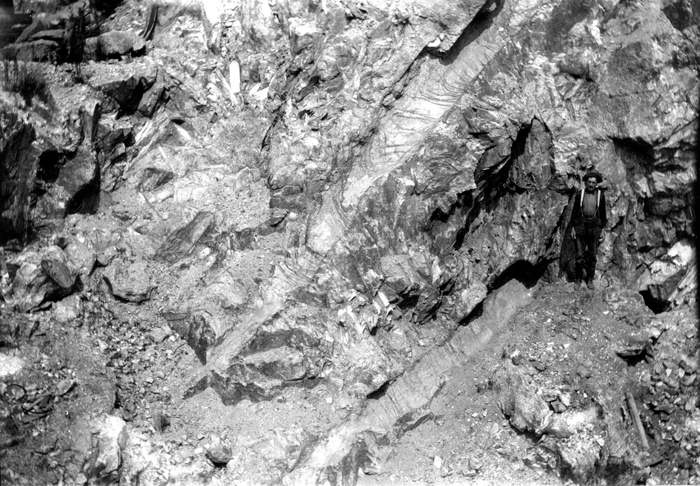
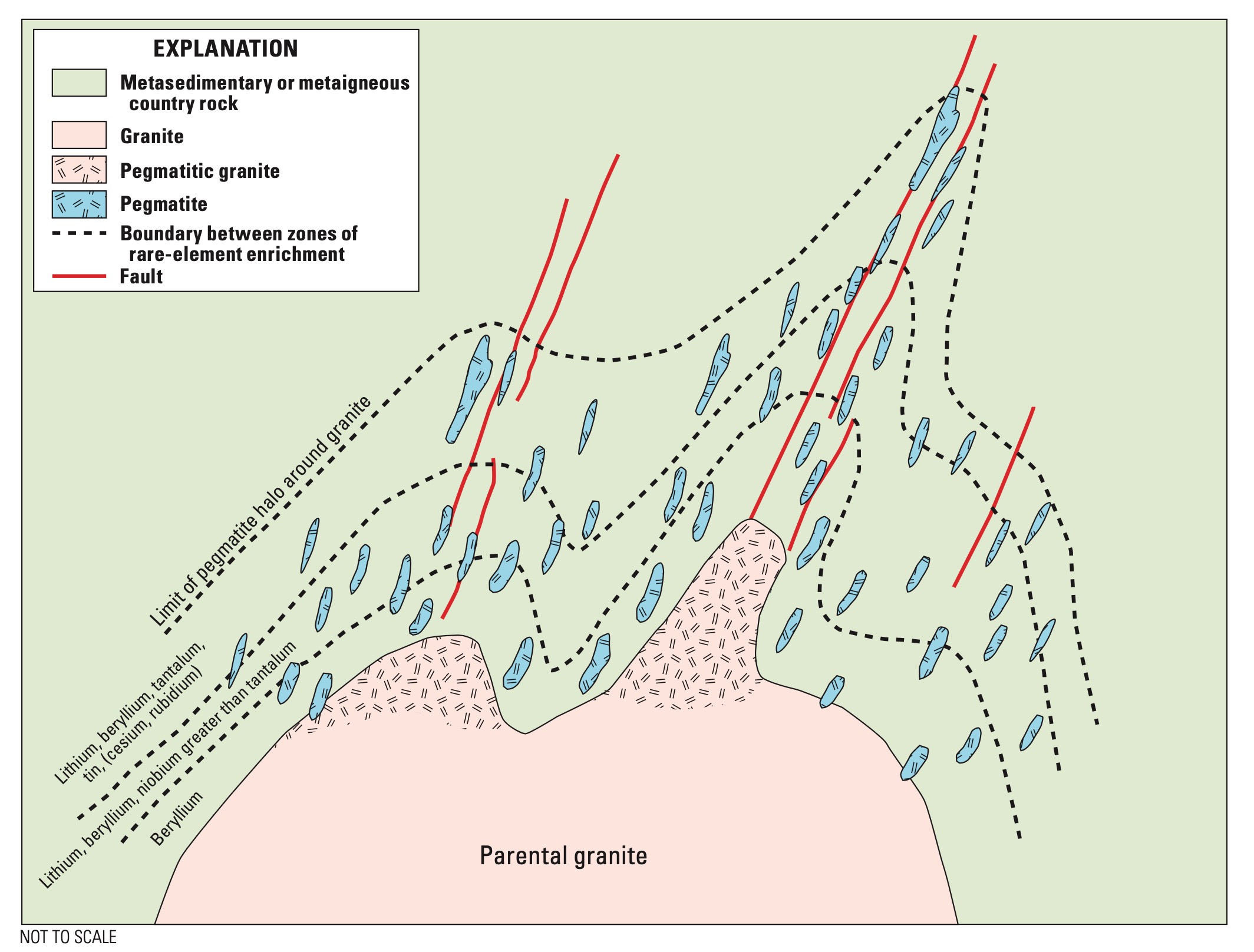
Foreland basins
Wherever mountains are formed in an orogen they have an isostatic effect on the adjacent plate, pushing the plate down. When this plate carries continental crust, a deep trough may be formed, called a foreland basin. Sedimentation in foreland basins is typically rapid, because a lot of material is eroded from the adjacent mountains. Initially these sediments are typically deposited by underwater flows of turbidity currents, but eventually, if sedimentation catches up with subsidence and fills the foreland basin to sea-level, turbidites may be overlain by shallow-marine sediments or river deposits.
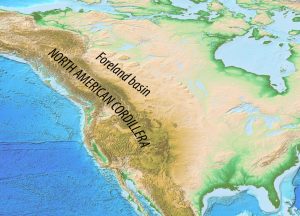
Foreland basins have a very characteristic wedge shape in cross-section, deepening steadily towards the mountains. A long-lived orogen like the North American Cordillera may incorporate slices of its own foreland basin as it grows.
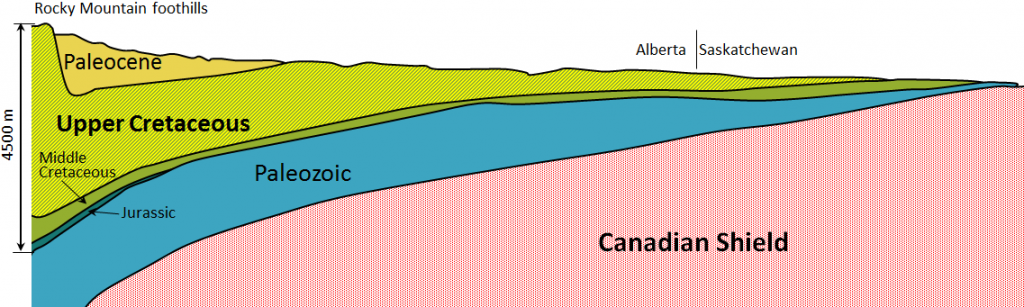
In various parts of the world, foreland basins contain major deposits of coal, or reservoirs of oil and natural gas. For example, in North America, the Paleozoic foreland basin of the Appalachian mountain range contains major coal deposits. The Mesozoic foreland basin of the Cordillera, known as the Western Canada Sedimentary Basin, overlies an older passive continental margin and contains large amounts of oil and natural gas. At least 400,000 oil and gas wells have been drilled in Alberta alone.
Cratons and supercontinents
Cratons
Continental areas that have been stable since about 500 Ma are known as cratons. Where igneous and metamorphic rocks are exposed at the surface, cratons are known as shields. Portions covered with sedimentary rock are known as continental platforms.
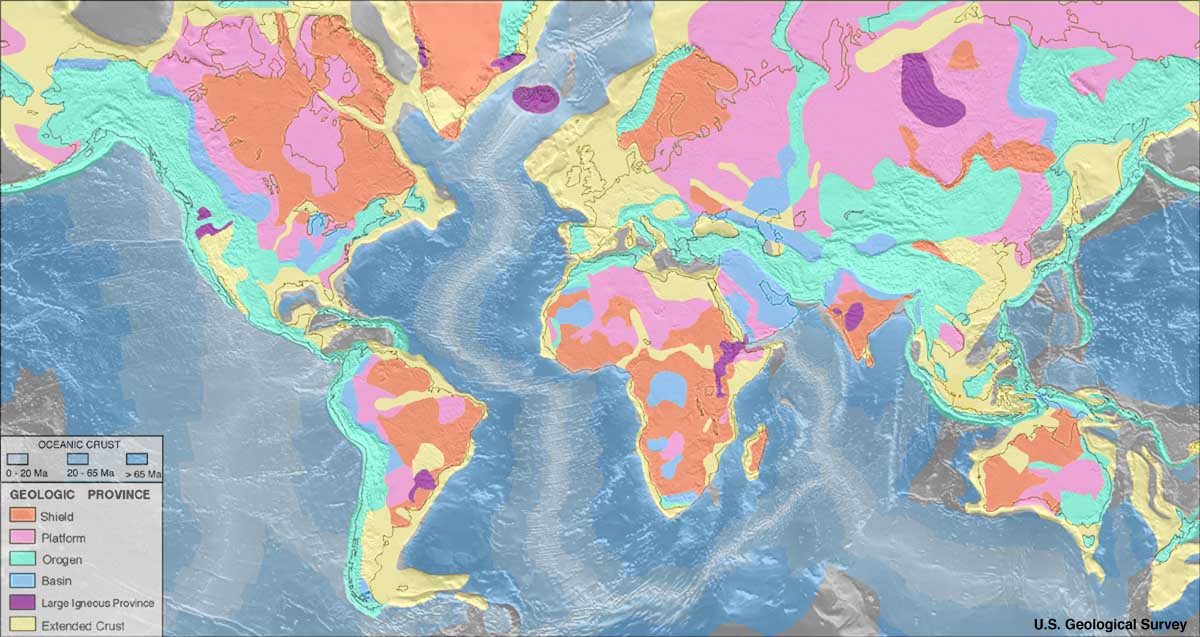
Large igneous provinces
Compared with plate margins, cratons in plate interiors are relatively slow-moving tectonic environments, but they are not without important resources. Hotspots in oceanic lithosphere (such as the one beneath Hawai’i) are mentioned in the section on plate movement. However, mantle plumes can also rise beneath continental crust, where they are responsible for the development of large igneous provinces: masses of magma producing intrusions and volcanoes within continents. Some large igneous provinces have eventually evolved into rifts. Others seem to have died out when the source of heat was removed.
Magmas in these provinces are predominantly mafic, because they are derived by partial melting of the mantle as it rises adiabatically and experiences lowered pressure. Mafic magma rises into the crust where it cools gradually. Crystals may grow and sink within the magma. Because the crystallizing minerals have different overall composition from the magma, there may be subtle variations in the composition of the magma. The settling crystals record these changes by forming layers on the floor of the magma chamber, rather like the layers typical of sedimentary rocks, but quite different in composition. Once cooled, these bodies of magma form layered igneous intrusions.
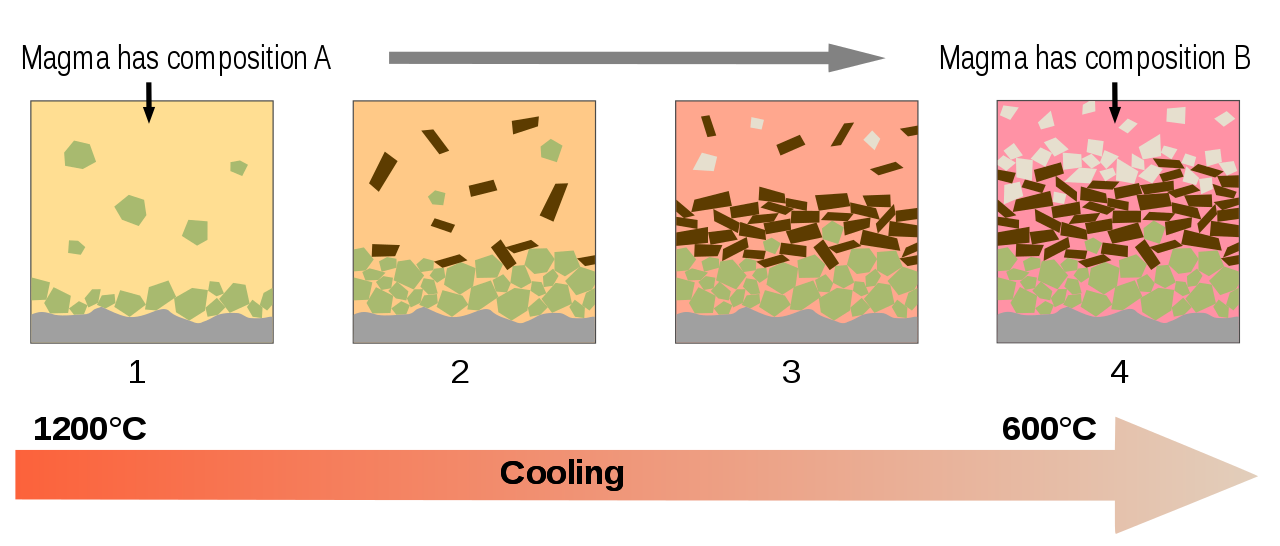
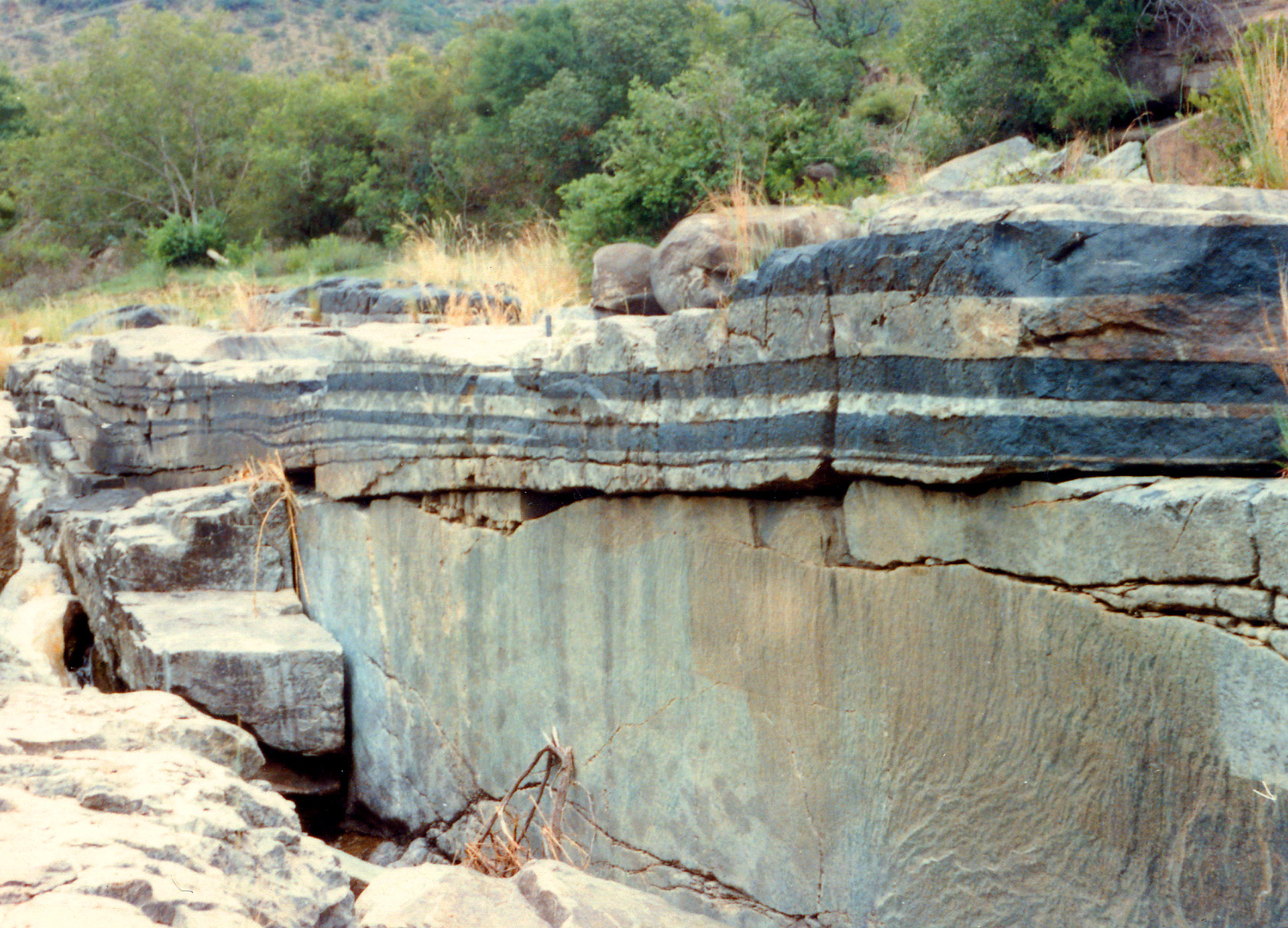
In some layered igneous intrusions, minerals such as chromite, a chromium oxide, are highly concentrated in dark layers. One example, the Bushveld intrusion in South Africa, contains over half of the Earth’s chromium reserves, together with very large reserves of platinum group elements. Chromium is an important component of many types of steel, as it both hardens the metal and makes it rust-resistant. Platinum group elements have major uses in catalytic converters (used to convert poisonous carbon monoxide to carbon dioxide in car exhaust systems) and in jewelry.
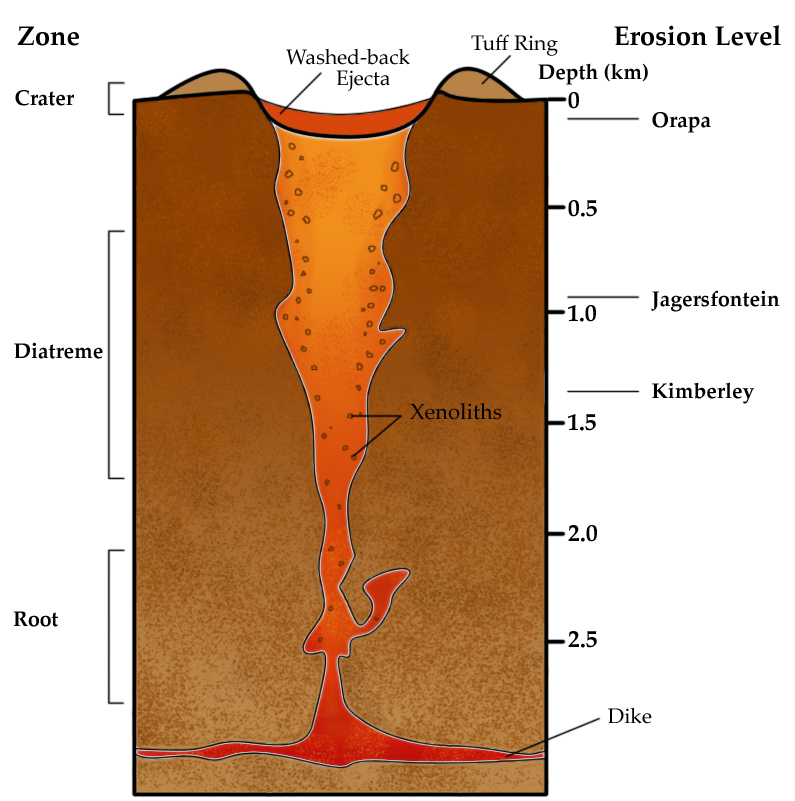
Another type of igneous rock that is associated with stable continental plate interiors is kimberlite. Kimberlite is a fragmental rock produced in violent volcanic eruptions that are clearly sourced from deep in the mantle, because the fragments include ultramafic rocks and minerals that can only form at very high pressure. They are humanity’s main source of diamonds. No kimberlite eruptions have ever been recorded in real time, but kimberlite pipes are known from most of the Earth’s continental areas, and their shape has been reconstructed from data collected during diamond mining operations. The most recent erupted in the Igwisi Hills in Tanzania at about 12 ka, young enough to have been observed by humans but too old for there to be any known historical record. The next youngest kimberlite occurred at about 30 Ma.
When not subject to igneous activity from mantle plumes, cratons typically show a history of gentle subsidence. Sedimentary strata may be deposited on the top of a craton, forming a continental platform, and relatively thin sedimentary basins may form in these areas. A notable example is the Proterozoic Athabasca Basin on the Canadian Shield, which is a major source of the element uranium. Uranium is present in small amounts throughout the shield, particularly in felsic igneous rocks. However, it may become concentrated in clastic sediments that were derived by erosion of the shield, particularly at the boundaries between oxidised and reduced sedimentary rocks. Uranium is mined by humans because of its radioactive properties. The more radioactive isotopes are concentrated for human uses. During the period 1945–1965 CE this was primarily for weapons. Subsequently, larger amounts of uranium have been extracted for electricity generation in nuclear reactors. The mining of uranium involves significant health hazards from radioactivity, which have been disporportionately borne by Indigenous communities over its relatively short history. Other environmental hazards from the use of uranium include accidents at nuclear facilities (including those at Chernobyl in Ukraine in 1986 and in Fukoshima in Japan as a result ot the Tohoku earthquake in 2011). An additional challenge is the disposal of nuclear waste: because of the long half-lives of uranium isotopes, reactor fuel remains radioactive long after the energy output has declined below what is useful for electricity generation. However, producing energy from uranium avoids the inevitable greenhouse gas impact associated with burning hydrocarbons. Balancing the environmental and health impacts of these different energy sources on the spheres of the Earth is a major challenge facing humanity.
Supercontinents and the history of the crust
Our review of plate tectonics has shown that the oceanic lithosphere, including the oceanic crust, is continuously being recycled into the asthenosphere and lower mantle by the process of subduction, which consumes lithosphere at the same rate that it is formed at spreading centres. As a result of this continuous recycling, no in-place ocean floor is older than about 200 Ma. The only fragments of older ocean floor are those preserved as ophiolites in orogens.
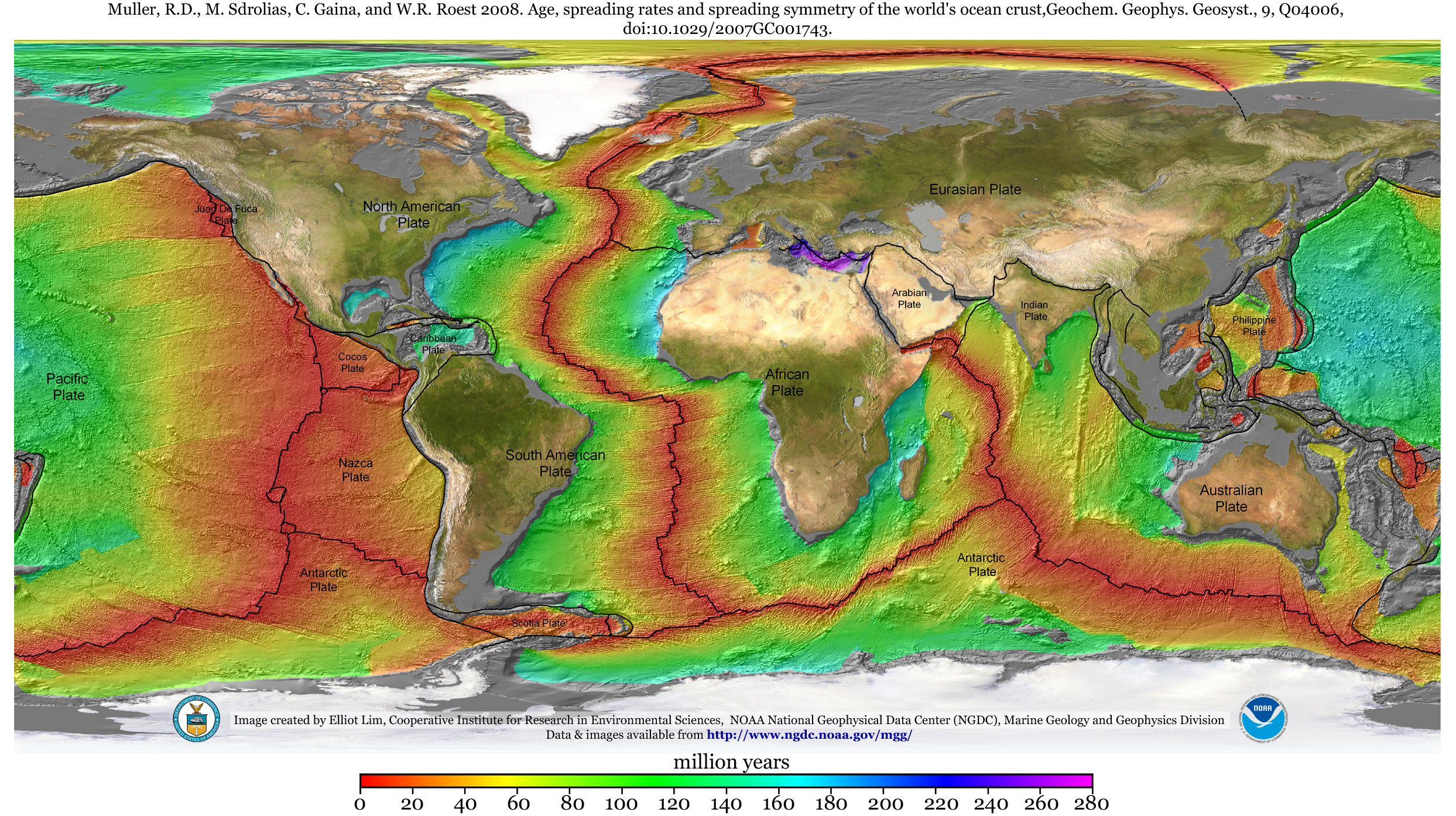
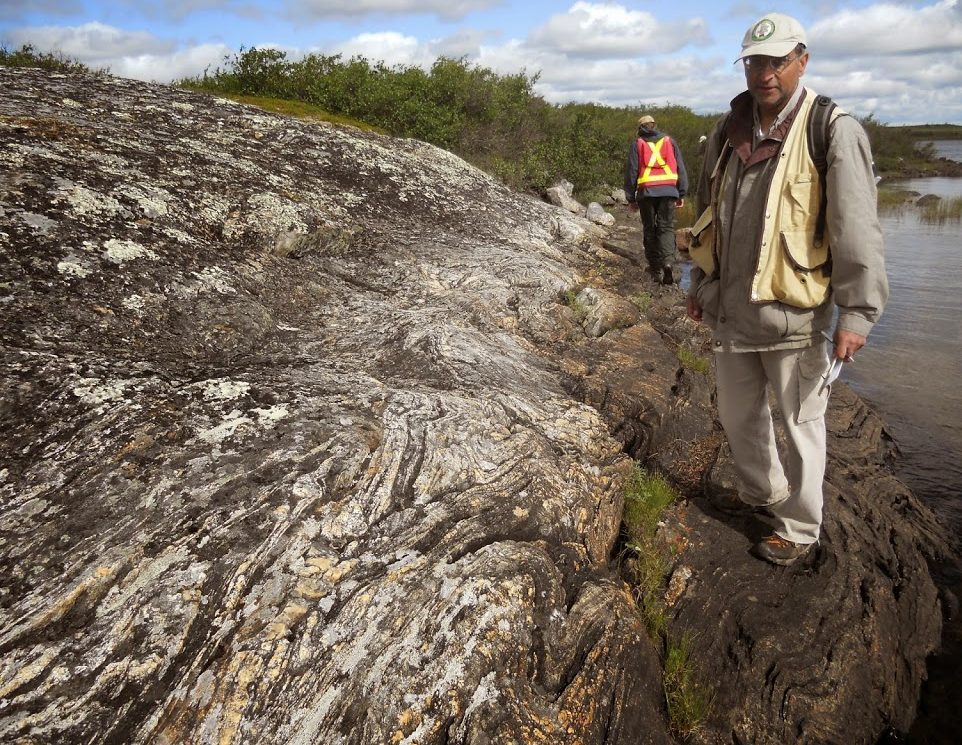
In contrast, continental crust, once formed, is never completely subducted. (In a few orogens we find evidence from high-pressure minerals that continental crust may have been pulled deeper than 100 km, but the ‘attempted subduction’ of continental crust only lasted a few million years before isostasy brought it back to the surface again. As a result, continental crust, once forms, remains at the surface indefinitely, and the continental crust contains a much longer (though more intermittent) record of Earth history, going back to about 4.0 Ga, the age of the Acasta Gneiss in the Northwest Territories of Canada.
The amount of continental crust has probably increased through geologic time, though whether this accumulation of continental crust was steady or irregular is a current controversy in Earth science. What is known, is that at various times in Earth history, the majority of the Earth’s continental crust has become clumped together in a single supercontinent.
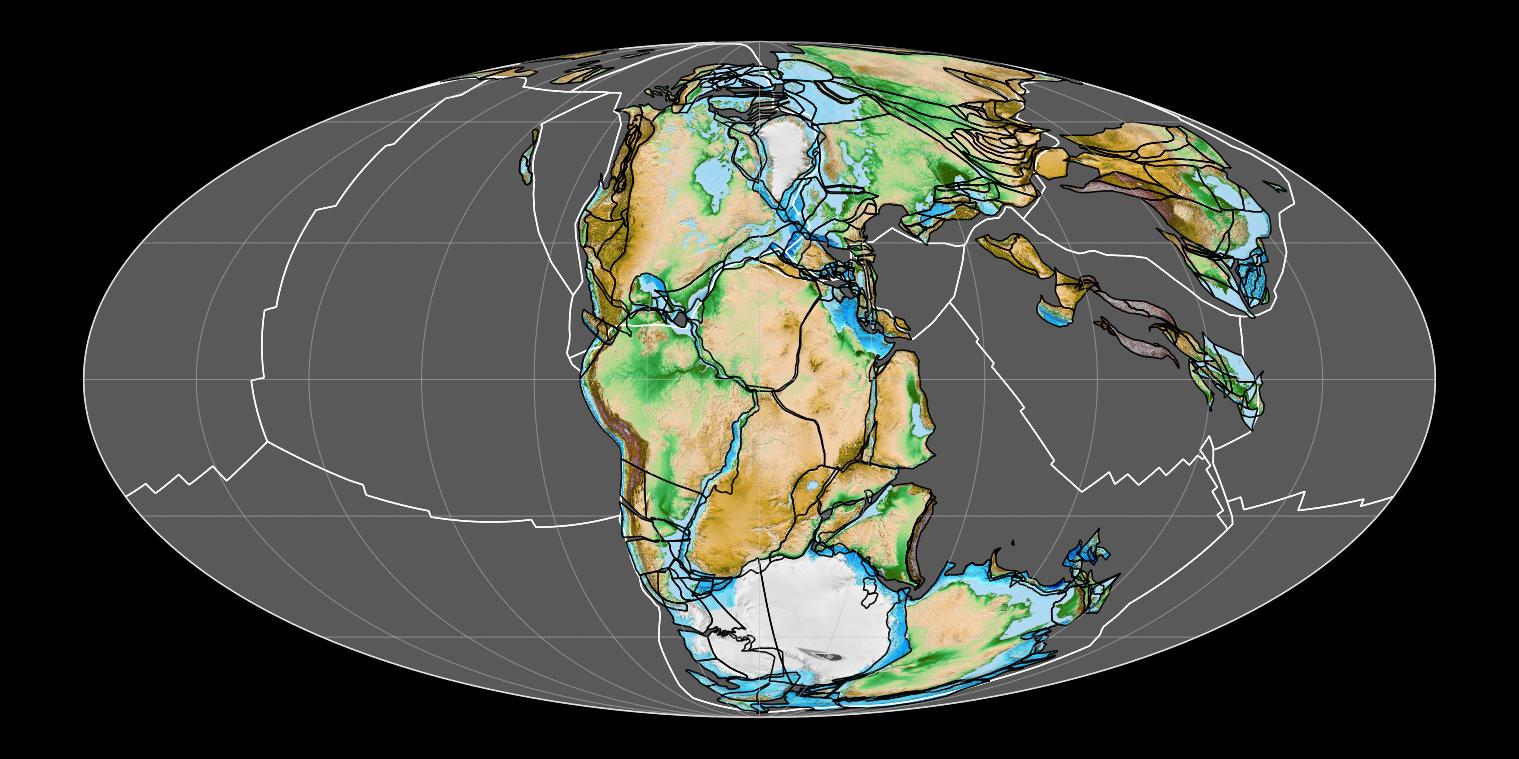
The most recent supercontinent is known as Pangea or Pangaea [2]. It existed from about 350 to about 200 Ma. Several mountain belts, including the Appalachian Orogen in North America and its continuations, the Caledonian and Variscan orogens in Europe, record the building of Pangea.
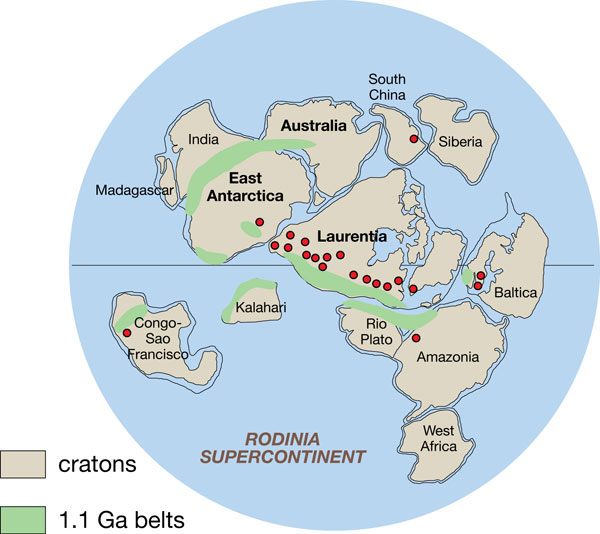
There is good evidence that most of the Earth’s continental crust was grouped together at around 1 Ga, or 1000 Ma, in a supercontinent that has been named Rodinia. A large orogenic belt along the east edge of the Canadian Shield, the Grenville Orogen, dates from this time, and has continuations in other modern continents including Europe and South America. It probably records continent-continent collisions that led to the formation of Rodinia.
Earlier supercontinents may have included Nuna at around 1.8 Ga in the early Proterozoic and Kenorland, around 2.7 Ga in the late Archean.
Before this time, there is a great deal of controversy about the nature of the tectonic system. There was undoubtedly a tectonic cycle in the Archean, but whether it was the same or different from modern plate tectonics is a matter of debate. There appear to be systematic differences in the characteristics of Archean rocks suggesting that the tectonic system was different. For example, Archean felsic intrusive rocks typically contain less potassium and more sodium than modern granites, and passive continental margins, characterized by thick accumulations of shallow-water shelf sediment, are unknown before about 3 Ga. The Archean Earth must have looked very different from the present-day Earth, but the exact nature of the Archean tectonic system is a subject of active research among Earth scientists.
- Most ophiolites were probably formed in smaller ocean basins like the Japan Sea and Phillippine Sea, rather than large basins like the Atlantic and Pacific ↵
- These two spellings are both derived from Greek words meaning 'all' (pan) and 'Earth' (Gaia). However, scientific terms usually use the Latin spellings of Greek words, following either classical Latin spelling as often used in the UK ('Gaea') or medieval religious Latin, commonly used in the USA ('Gea'). ↵
Describes tectonic motion that is mostly horizontal and parallel to a tectonic boundary
Near-horizontal movement between two blocks separated by a fault.
The boundary between two plates moving towards one another. Convergent boundaries are the sites of subduction of one plate beneath the other.
The solid Earth
Materials such as fossil fuels and minerals which do not form at a rate fast enough to replace their consumption by humans.
Materials or energy supplies, useful to humans, that are replenished at a rate comparable to that of human consumption.
Grade (mineral deposits): The concentration of a desired element in rocks
A dense yellow metal element (Au), atomic number 79.
Naturally occurring accumulations of useful material in concentrations and amounts that make extraction by humans currently or potentially feasible.
Resources that can be exploited economically under present conditions.
Fine-grained sedimentary rock which holds a large proportion of hydrocarbons.
Natural gas is trapped within fine-grained sedimentary rock.
A plate boundary where plates are moving apart, away from the boundary
Elevated regions on the ocean floor centred on divergent plate boundaries.
Concentrations of minerals deposited by hot groundwater.
Openings, typically on the ocean floor, from which hot water or steam is able to escape.
Sections of mafic or ultramafic rock from oceanic lithosphere that have been uplifted on land.
A type of mineral deposit formed by hydrothermal fluids flowing through igneous rock, typically rich in copper and other elements
A localized region at the Earth's surface located above an unusually hot part of the mantle.
Energy originating from within the Earth, either from radioactive decay or from the orignal heat trapped at the planet's formation
An era in the Phanerozoic Eon of Earth history, from about 200 Ma to about 65 Ma
Stretching of the lithosphere along a belt marked by faults
A fracture in Earth’s crust where bodies of rock may slide past each other.
The behaviour of upper layers of the Earth as if floating on the more deformable Asthenosphere below; lower density material corresponds to higher elevations.
Mohorovičić Discontinuity: The boundary at the base of the Earth’s crust where seismic P-waves abruptly increase in velocity.
A boundary typically between 50 and 250 km deep within the Mantle, separating rigid plates above from slowly deforming material below
A change to a system is adiabatic no heat energy is gained or lost during the change
Describes a statistical distribution that has two peaks or modes
Of silicate minerals and rocks, having large amounts of magnesium and iron; mafic rocks have silica contents of 45–50%.
Igneous rock or magma with more than about 65% silica. Felsic rocks typically contain quartz
with potassium and/or sodium feldspar.
A continental margin that does not coincide with a a plate boundary.
An area of shallow sea (<200 m) at the edge of a continent, underlain by continental crust.
Liquid Petroleum; organic matter that has undergone burial and subsequently been transformed into hydrocarbon-rich liquid.
Hydrocarbon-rich gas derived from sedimentary organic matter.
Areas on the surface of the Geosphere where petroleum (oil or natural gas) escapes.
Hydrocarbon deposits where a porous reservoir rock has enough permeability to yield oil or natural gas when penetrated by drilling.
A process of pumping pressurized fluid into rock, causing fractures that increase permeability
Unconventional hydrocarbons are those contained in low-permeability rocks, that do not flow out of the rocks without intervention
A sediment deposit formed from the flow of a channel into a standing water body.
Carbon-rich material formed from compressed plant remains; a first stage in coal formation.
A layer, or bed, of coal
Carbon-rich sedimentary rock formed from solidified organic material that originated as peat
Carbon-rich substances found in the geosphere and formed from the remains of past-living organisms. Fossil fuels contain sufficient concentration of reduced carbon to produce usable heat when burnt in air.
Mineral matter deposited by groundwater in the pore space of a rock.
An anthropogenic mixture of cement and coarse sediment (sand or gravel), used in
construction.
Layers of iron-rich minerals and chert that were particularly common in the Proterozoic Eon
A plate boundary where relative plate motion is parallel to the boundary.
A fault where the side opposite to an observer appears to be moving to the observer's right.
Fault motion such that the far wall of a fault appears displaced to the observer's left
Bends along the sides of a fault where both sides push slightly together.
A bend on a fault where the two walls of the fault move slightly apart
A sedimentary basin formed as at releasing bend on a fault such as a transform fault, where motion is near-horizontal.
A depression in the lithosphere created by the isostatic effect of an adjacent mountain belt.
A blue chain-silicate mineral formed during the recrystallization of ferromagnesian minerals and feldspar under high-pressure conditions.
A metamorphic rock formed at high pressure and relatively low temperature; named for the presence of the blue sodium-bearing silicate mineral glaucophane.
A group of mainly ferromagnesian single-chain silicate minerals common in mafic rocks
A metamorphic rock formed at high pressure and moderate to high temperature; components of feldspar become dissolved in red garnet and green pyroxene
The layer within the Earth or other inner planet that overlies the core and makes up the majority of the planet, composed chiefly of magnesium- and iron-rich silicates
A chain of volcanoes that lies above a downgoing slab of lithosphere at a subduction zone
An igneous rock or magma with between 52% and 65% silica
Silicate minerals that contain large amounts of magnesium and/or iron, typically in solid solution, and a relatively low amount of silica.
Changes in the composition of magma due to the removal of certain chemical components, often as a result of mineral settling.
A continental margin that coincides with a plate boundary
The fringe of a continent were it shares a boundary with oceanic crust.
A process whereby magma solidifies within the Geosphere.
Copper sulphide deposits typically associated with porphyritic igneous rock.
Igneous rocks with both fine and coarse mineral grains.
A gently sloping fault where the hanging wall has moved up the slope of the fault over the footwall.
The block of rock that lies above a dipping fault surface.
A principle in geology which states that the younger strata formed above older strata.
A mountain-building process that occurs as crust shortens during the convergence of the lithosphere.
A mountain belt formed by shortening of the crust.
A mountain range at the western edge of North or South America, formed by plate convergence.
A mountain chain formed where continental crust enters a subduction zone, coming into contact with another continent or an oceanic volcanic arc.
A process of orogenesis that occurs where a plate carrying continental crust enters a subduction zone at an oceanic volcanic arc. Continental crust undergoes shortening and thickening, producing mountains.
A process of orogenesis that occurs where two converging plates both carry continental crust. Continental crust undergoes shortening and thickening, typically producing high mountains.
Large igneous intrusions
Element 3, the lightest metallic element
A turbulent flow of sediment-rich water moving down slope under clearer water due to the action of gravity.
The core of a continent that has been stable since at least 500 Ma
Areas of cratons (stable continental crust) where Archean or Proterozoic metamorphic and igneous rocks are exposed.
Portions of a craton that have been covered in sedimentary rock.
Regions of the Earth where large amounts of magma (usually mafic) have been generated in a geologically short time.
A belt of stretched lithosphere, typically marked by one or more fault-bounded valleys
Bodies of mafic magma that cooled within the Geosphere, in which layers of different composition have formed.
Chromium oxide, a dark mineral often concentrated in the layers of layered igneous intrusions.
Formula Cr2O3
Six metallic elements with similar properties: Ruthenium, Rhodium, Palladium, Osmium, Iridium and Platinum.
Ultramafic tuff produced by explosive volcanic eruptions sourced from the mantle; the main source of diamonds.
A crystalline mineral form of elemental carbon the hardest known mineral.
Large cylindrical structures preserving ultramafic tephra sourced from the mantle; the main source of diamond.
The amount of time required for a mass of a particular isotope to decay until only 50% of the parent isotope remains.
Isotopes of an element are atoms that have the same atomic number but different atomic mass, because of different numbers of neutrons in the nucleus
A large conglomeration of continental lithosphere, typically including the majority of continental crust on Earth at a given time.
Pangea (UK: Pangaea): A supercontinent formed during the late Paleozoic Era and dispersed during the Mesozoic and Cenozoic eras
An ancient supercontinent preceding Pangaea. Rodinia existed during the Proterozoic Eon.
A supercontinent which may have formed 1.8 billion years ago in the early Proterozoic Eon.
An early supercontinent that may have formed around 2.7 Ga in the late Archean.
An eon early in Earth history prior to the Proterozoic from ~4 Ga to 2.5 Ga