17 What the Geosphere is Made of: Minerals
Learning Outcomes
By the end of this section you should be able to:
- State the differences in major element abundances between parts of the Geosphere
- Define minerals and use simple methods to identify mineral properties
- Describe the main groups of silicate minerals
- Describe selected examples of non-silicate minerals
The next sections of the course are about the Geosphere. As with the other spheres, we will begin with a look at what elements go in to the Geosphere. However, unlike the other spheres, the list of elements that are found in the Geosphere is long, and the composition of the Geosphere is very varied. We will therefore just focus on what is most abundant. Bear in mind that there are about 90 naturally occurring elements in the periodic table, and every one of them is found in the Geosphere!
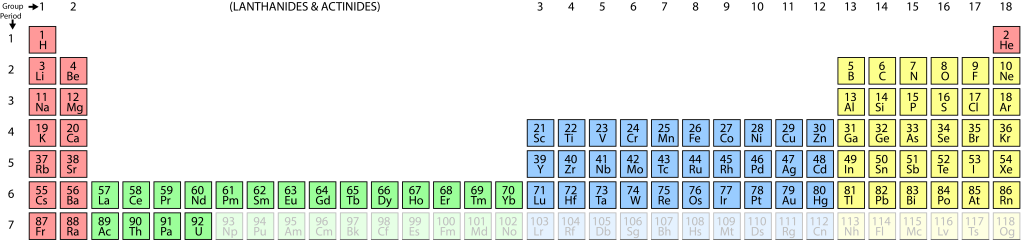
Atomic symbols
In some cases we will use standard symbols to represent elements, as shown in the image of the periodic table. Most of these are quite logical: for example Si represents silicon. However, a few of them are based on older, Latin names for elements, and are not obvious at first. The most important examples for us are…
- Fe is Iron
- Na is Sodium
- K is Potassium
- Pb is Lead[1]
- Ag is Silver
- Au is Gold
Elements in the Geosphere
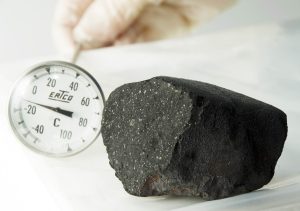
Our knowledge of the abundance of elements in the Geosphere, the solid Earth, is somewhat limited, because the deepest drilling by humans penetrates only just over 12 km into the Earth, a tiny fraction (0.2%) of the Earth’s total radius (6370 km). If we look at samples brought up from the mantle by volcanic eruptions of kimberlite, we can perhaps ‘see’ material from a few hundred (perhaps even 450) kilometres down, but this is still well under 10% of the Earth’s radius, and the samples we get are probably not from very typical mantle rocks. Our information about the deep interior has to be based on remote sensing methods, particularly the Earth’s gravity, magnetic field, and the behaviour of seismic (earthquake) waves as they pass through the interior. Based on these properties, it is possible to infer that the Earth’s interior, like that of the other terrestrial planets, can be divided into
- a central core, consisting mainly of iron;
- a mantle, the largest part by volume, consisting of magnesium and iron silicate minerals;
- a very diverse, but relatively thin crust.
Because of the impossibility of sampling the deep interior of the Earth, our best estimates of the overall composition of our planet are actually based mainly on chondrite meteorites, thought to represent material left over from the origin of the solar system, and therefore representative of what went into the terrestrial planets Mercury, Venus, Earth, Mars, and the Moon.
Based on all these lines of evidence, the most abundant elements in our planet are probably as follows:
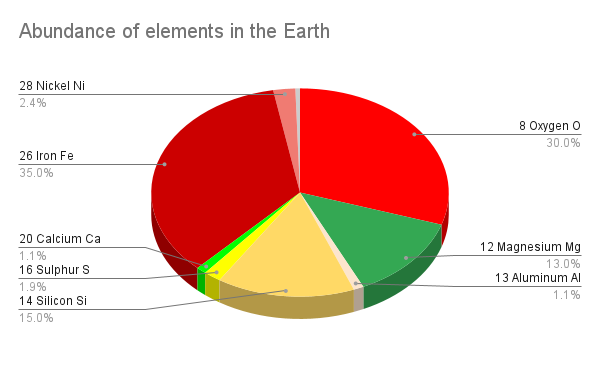
- Iron (Fe, ~35%)
- Oxygen (O, ~30%)
- Silicon (Si, ~15%)
- Magnesium (Mg, ~13%)
- Nickel (Ni, ~2.4%)
- Sulphur (S, ~1.9%)
- Calcium (Ca, ~1.1%)
- Aluminum[2] (Al, ~1.1%)
- Everything else (<1%)
However, these elements are unevenly distributed within the Geosphere. The Earth’s core is mostly iron, with nickel as the second most abundant element, so much of the Earth’s iron and nickel are locked up in the core. The mantle also contains some iron, but it also has abundant oxygen, silicon, and magnesium, with all the other elements listed in the table in lesser amounts. However, over the Earth’s 4.6 Ga history, silicon and oxygen, toether with calcium, aluminum, and many of the elements included in “everything else” have been preferentially concentrated in the crust, in a process of planetary differentiation.
The same overall distributions probably occur in the other terrestrial planets (though our knowledge of their interiors is even more sketchy, of course). However, each of these planets has an iron-dominated core, and a mantle with a composition similar to Earth’s, their crusts are wildly different from one another, because each planet has its own rock cycle, and it is the rock cycle that has formed the crust.
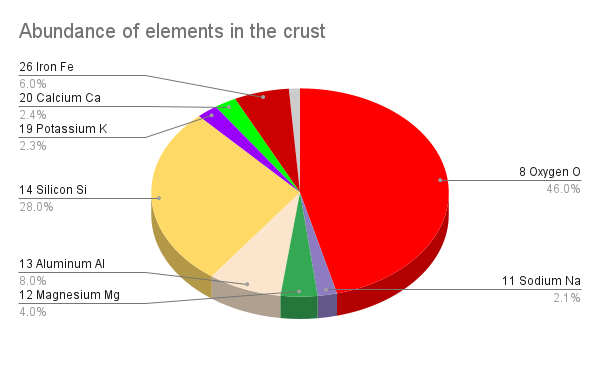
When we look at the Earth’s crust we see that there’s much less iron, magnesium, nickel and sulfur, and much more of almost everything else.
- Oxygen (O, ~46%)
- Silicon (Si, ~26%)
- Aluminum (Al, 8%)
- Iron (Fe, ~6%)
- Magnesium (Mg, ~4%)
- Calcium (Ca, ~2.4%)
- Potassium (K, 2.3%)
- Sodium (Na 2.1%)
- Everything else (~1%)
Minerals
Most of these elements occur in solid chemical substances called minerals. Each mineral has either a fixed composition, or varies between certain fixed limits. Most parts of the Geosphere are made of mixtures of minerals, called rocks. In this section we will look at the properties of minerals. Often, recognizing minerals is an important step towards understanding how a rock formed.
Scientific definition of a mineral
To be classified as a mineral in Earth science, a substance must satisfy the following criteria.
- Solid
- Naturally occurring
- Inorganic
- Fixed (or limited range of) chemical composition
- Crystalline structure: atoms are arranged in a regularly repeating 3-dimensional pattern called a crystal lattice
By this scientific definition, about 6000 discrete minerals are known. This definition has some unexpected consequences!
- Ice is a mineral (fits all 5 criteria)
- Coal is not a mineral because it is organic, and has no crystalline structure.
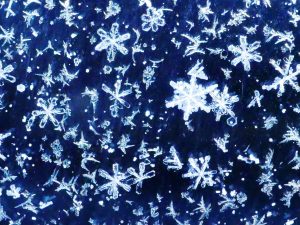
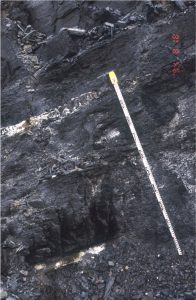
In other realms (e.g. law) the term mineral has different definitions. For example,
- Coal is a legal mineral in most jurisdictions;
- Ice is not legally a mineral.
Rocks vs. minerals
In contrast with minerals, rocks are mostly naturally occurring mixtures of minerals. Unlike minerals, rocks don’t always naturally fall into discrete categories. Although Earth scientists classify rocks using a variety of names, their compositions are continuously variable, and borderline cases are quite common.
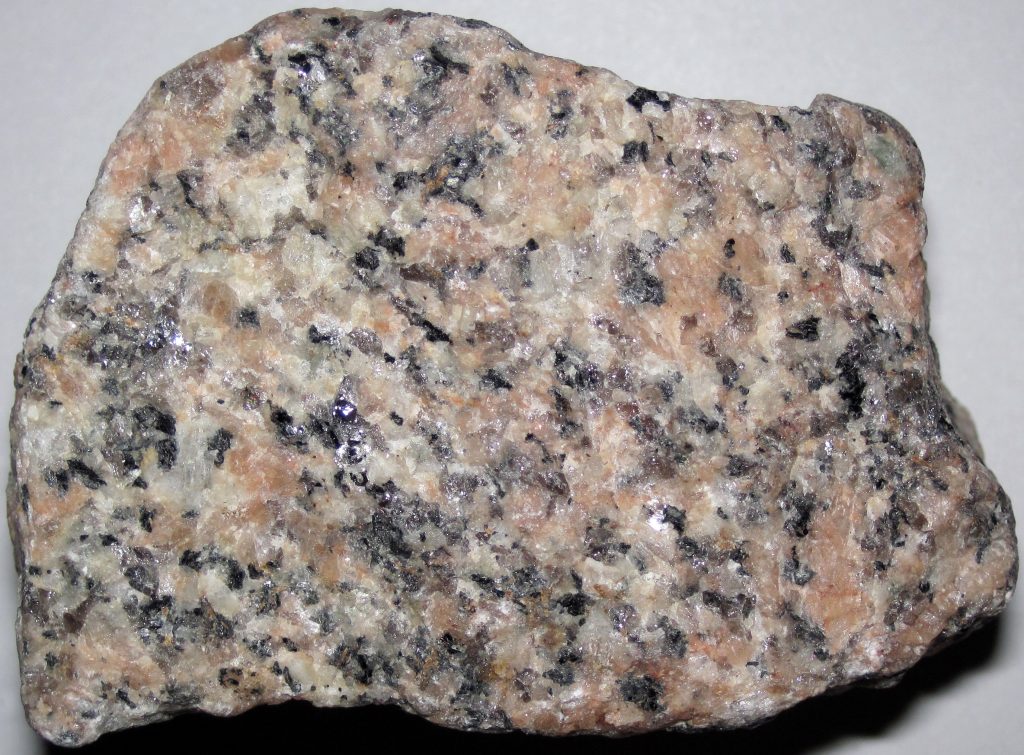
For example, a typical rock such as granite contains several minerals. In the case of granite these are commonly:
Rocks may also contain non-mineral materials, for example:
- Organic matter such as carbon-based polymers. Coal is a rock made largely of non-mineral organic matter.
- Volcanic glass – effectively a supercooled liquid in which the atoms occur in a chaotic arrangement that has no crystal lattice.
Crystal lattices
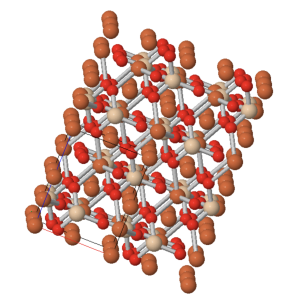
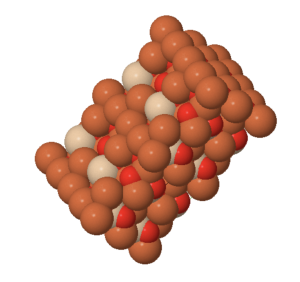
Minerals are dstinguished by having a structure in which atoms are linked in 3 dimensions forming a crystal lattice. Although the term ‘crystal’ is used in common speech to describe a mineral grain with flat outside faces, all minerals are crystalline. The large flat surfaces of ‘crystals’ certainly reflect the symmetry of the internal lattice, but the are not necessary for the mineral to be called crystalline. Typically, the flat outside faces develop in relatively rare circumstances when mineral crystals grow part-way into fluid-filled spaces within the Geosphere. In most cases, crystals grow until they collide with other crystals. Under these circumstances, smooth outside faces don’t usually form, but the internal arrangement of the atoms still reflects the crystalline structure that is characteristic of each mineral.
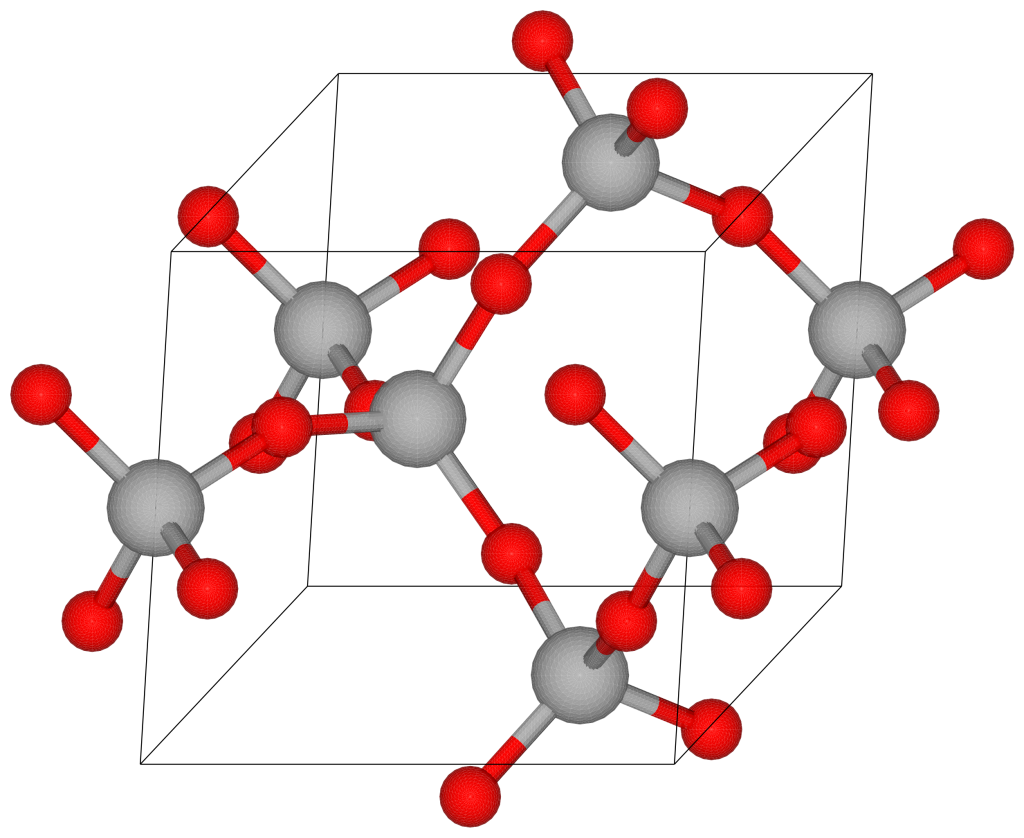
It’s important to understand that there aren’t really molecules in most crystals. Although we can write a formula like SiO2 for the mineral quartz, there are no SiO2 molecules in solid quartz; the silicon and oxygen atoms are bonded in a continuous crystal lattice. The formula merely expresses the fact that there are two oxygen atoms for every on silicon atom.
Three main types of bonding between atoms occur in natural crystals.
Ionic bonds
The quantum mechanics of electrons makes certain configurations stable. For most of the elements we are interested in, 8 electrons in the outer shell surrounding an atom is a stable configuration. As a result, elements like sodium and potassium, with a single electron in the outer shell, can easily lose that electron to become a positively charged ion. Conversely, atoms like chlorine, that have 7 electrons in the outer shell, can easily achieve stability by gaining an electron to form a negatively charged chloride ion.
Ions
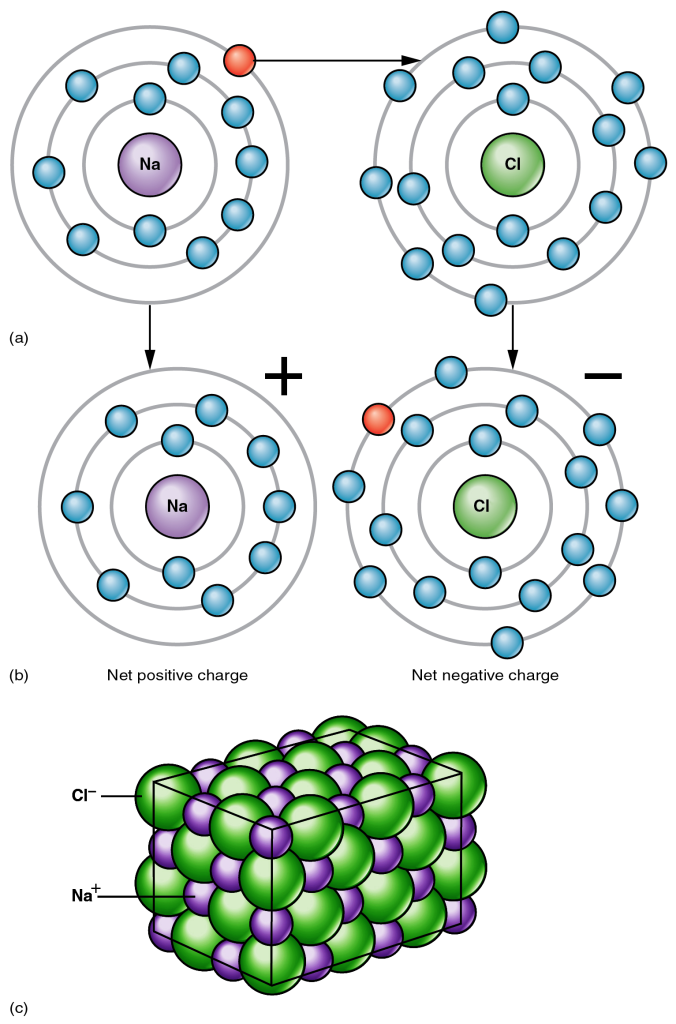
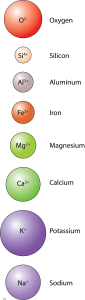
Hence, if a sodium atom is brought in contact with a chlorine atom, an electron tends to be transferred from sodium to chlorine, producing two ions, each with a stable eight electrons in their outer shell. The sodium ion carries a positive electric charge and the chloride ion has a negative charge. Because unlike charges attract each other, the two atoms tend to stick together. If many sodium and chloride ions are available, they assemble into a cubic lattice, in which the geometry is determined by the sizes of the ions.
The sizes of ions are partly determined by the number of electrons in the atom, but the electric charge is much more important. Negative ions have extra negatively charged electrons, so those electrons tend to be held more loosely by the positively charged nucleus. Negative ions, mostly of non-metallic elements, are therefore large, whereas positively charged ions (which are mostly metals) tend to be small.
Metallic bonds
A second class of bonding occurs in crystals of metals that are native elements:- minerals that contain only one kind of atom. Examples are copper and gold.
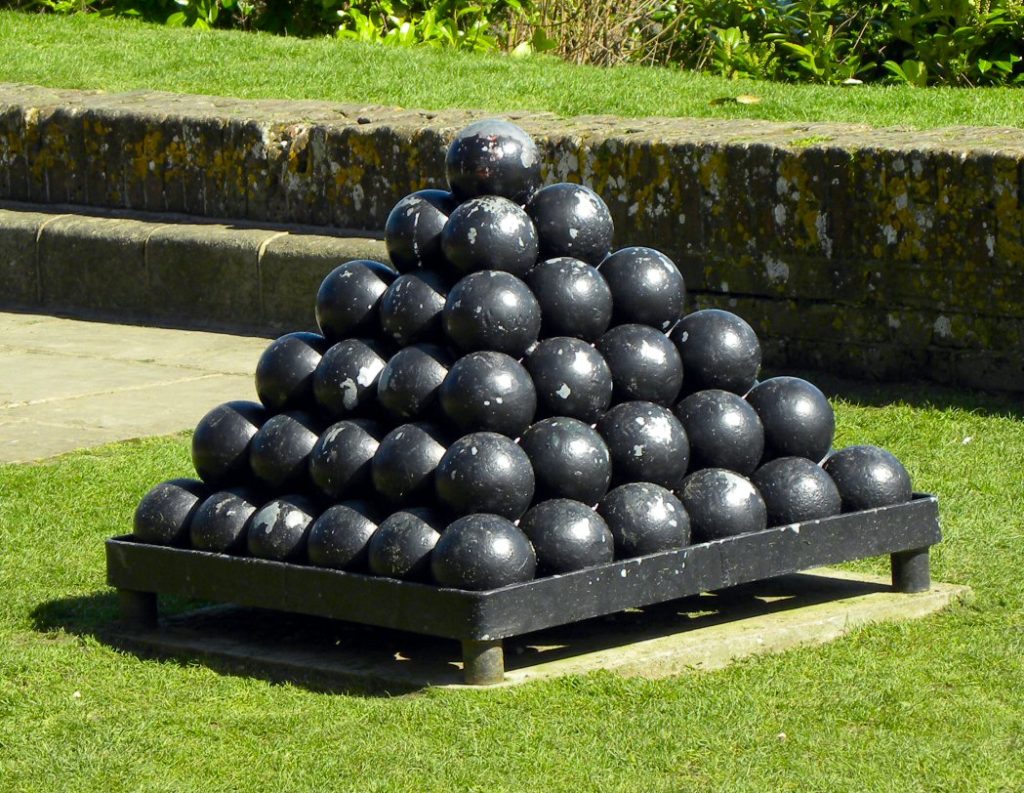
In these minerals, the outer, loosely held electrons associated with many atoms can merge to form a continuous electron cloud, effectively a single, merged electron shell, that holds the atoms together in a densely packed arrangement, usually with cubic or hexagonal symmetry.
Covalent bonds
A third type of bonding involves sharing of electrons between adjacent atoms. A common example occurs in silicate minerals. Silicon atoms, like carbon atoms, have four electrons in their outer shell, whereas eight electrons is the stable configuration. In many silicate minerals, silicon atoms share electrons from adjacent oxygen atoms so as to fill that outer shell. The oxygen atoms may aquire extra electrons to fill their outer shells too, to produce SiO42- groups known as silicate tetrahedra.
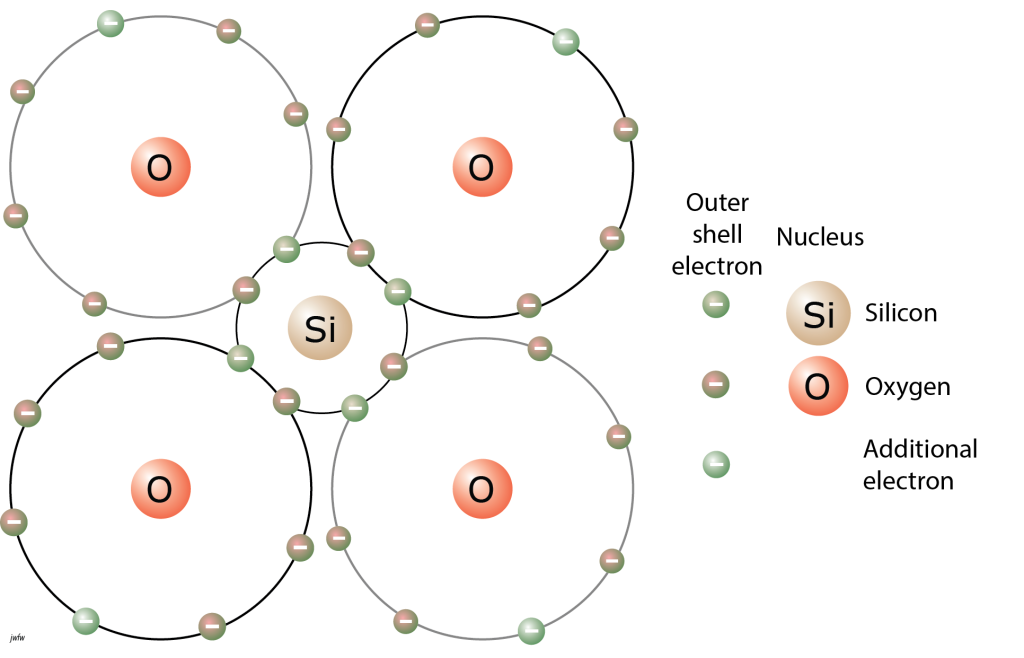
The bonds in diamond are entirely covalent, contributing to the great hardness of diamond, the hardest mineral known.
Polymorphs
Carbon forms the mineral diamond by covalent bonding. By sharing electrons with four adjacent carbon atoms, each carbon atom fills its outer shell with eight electrons, and the carbon becomes bonded into a strong 3-D lattice where each atom is surrounded by four others. The resulting structure produces the hardest mineral known. Carbon can exist in other forms too. In an alternative arrangement of atoms, carbon atoms are linked together in 2-dimensional sheets of hexagons. Only very weak bonds join the sheets to one another, producing a very soft mineral: graphite.
![]() |
![]() |
![]() |
![]() |
Both graphite and diamond have the same chemical formula — just C — but they are different minerals because they have different atomic structure. They are known as polymorphs[3].
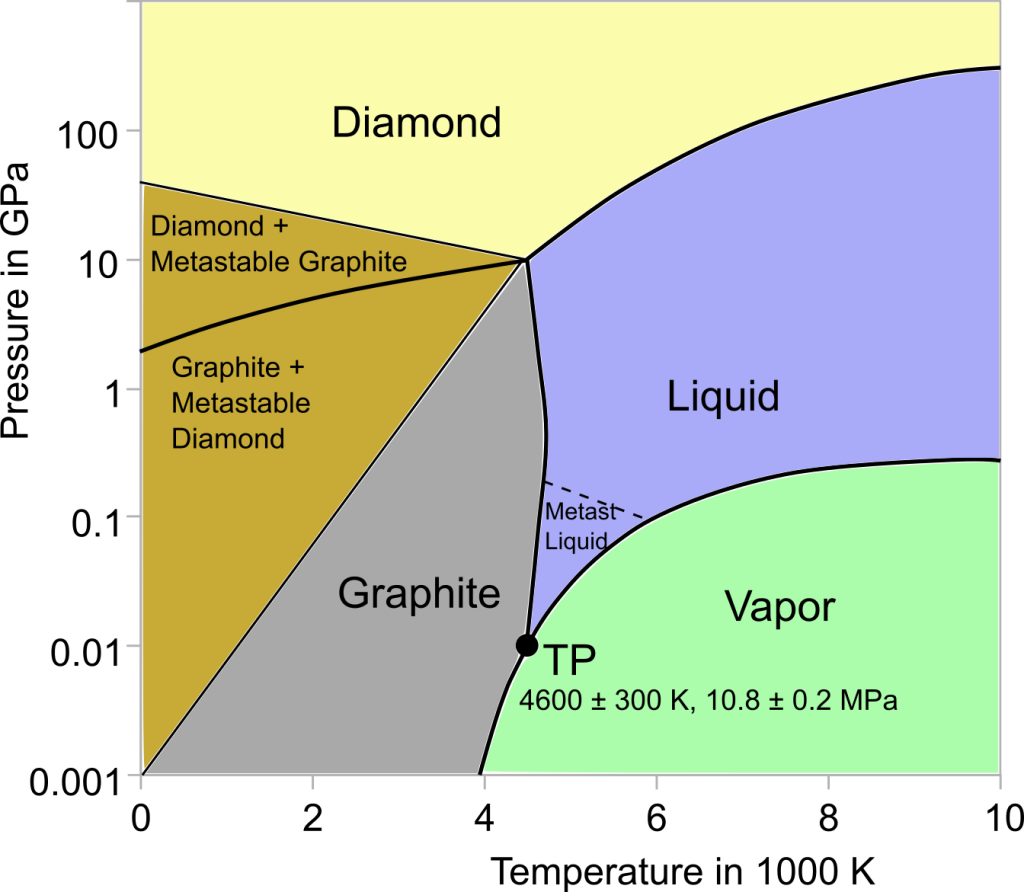
Polymorphs of minerals can provide information about conditions within the Earth, because each polymorph is stable under different conditions. The diagram is an example of a phase diagram, The axes of the graph are temperature and pressure. The diagram shows the stability regions of diamond and graphite, as well as liquid carbon and carbon gas. Each of these four forms of carbon is known as a phase. Phases are parts of a system that have different structure and are separated from one another by distinct boundaries.
Diamond is stable under conditions of very high pressure, more than 1 GPa (one gigapascal), such as are found in the mantle. Graphite is stable at lower pressures, including at the Earth’s surface where pressure is measured in just kilopascals. As you might expect, the liquid and vapour forms of carbon occur at high temperature.
How then is it possible for diamonds to survive at the Earth’s surface? The answer is that diamonds are metastable – given enough input energy to break the covalent bonds, the carbon atoms would reassemble into graphite, but starting the reaction takes a lot of energy, so diamonds are able to survive outside their field of stability.
Identifying minerals: mineral properties
In common rock types that form the majority of the Earth’s crust, it’s possible to identify minerals using simple physical properties, if the texture of the rock is coarse enough for the mineral grains to be clearly distinguishable.
Optical properties
Colour
Colour[5] is perhaps the most obvious of mineral properties, but it is not always the most helpful. Some minerals do have a distinctive colour, but in many other cases colour is determined by tiny amounts of impurities. Hence, the common mineral quartz can take on many different colours, some of which have their own names and are valued as semi-precious gemstones.
![]() |
![]() |
![]() |
![]() |
![]() |
![]() |
Despite these many names, all have the same crystal properties and almost exactly the same chemical composition. To a mineralogist, they are all quartz.
Lustre
![]() |
![]() |
![]() |
Lustre (Luster in the United States) describes the quality of light that reflects from the surface of a mineral. Many mineral surfaces reflect part of the light that strikes them, somewhat like glass. Such minerals are said to show glassy or vitreous lustre. Quartz is a common mineral that shows glassy lustre. Another common type of reflectivity is that shown by metals, which reflect most of the light striking their very opaque surfaces. Such lustre is described as metallic. The minerals pyrite and galena, sulfides respectively of iron and lead, show metallic lustre. Unreflective minerals are said to have earthy or dull lustre.
Streak
Although colour is often misleading as a mineral property, for some minerals the colour of a fine powder is distinctive. The iron oxide hematite (formula Fe2O3) ranges in colour and lustre from black and metallic to red and dull. However, finely powedered hematite always appears reddish in colour. The easiest way to test the colour of a powdered mineral is to draw a line with it on an unglazed porcelain tile, known as a streak plate. The colour is known as the streak of the mineral.
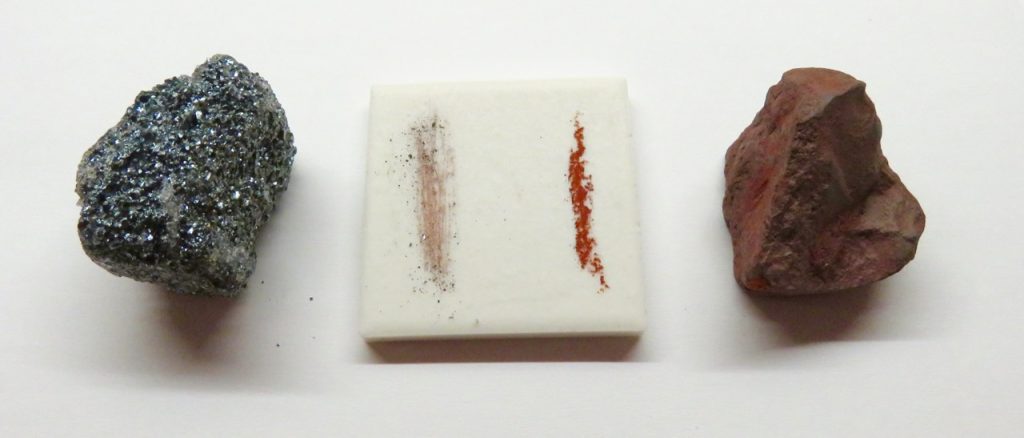
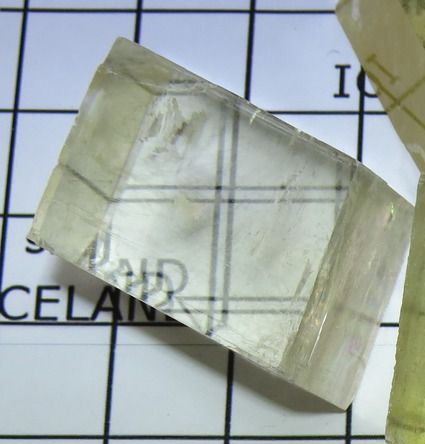
Other optical properties
Several other optical properties characterize particular minerals. Many are used for the identification of minerals under the microscope in the study of optical mineralogy. Here we mention just one, birefringence. The mineral calcite, the most common form of calcium carbonate CaCO3, when in clear crystals (sometimes known as iceland spar), calcite has the property of splitting light into two polarized beams that are refracted (bent) by different amounts, producing a double image of anything viewed through the crystal.
Crystal properties
Because each mineral has its own distinctive crystal structure, properties related to that structure can be very useful for distinguishing minerals.
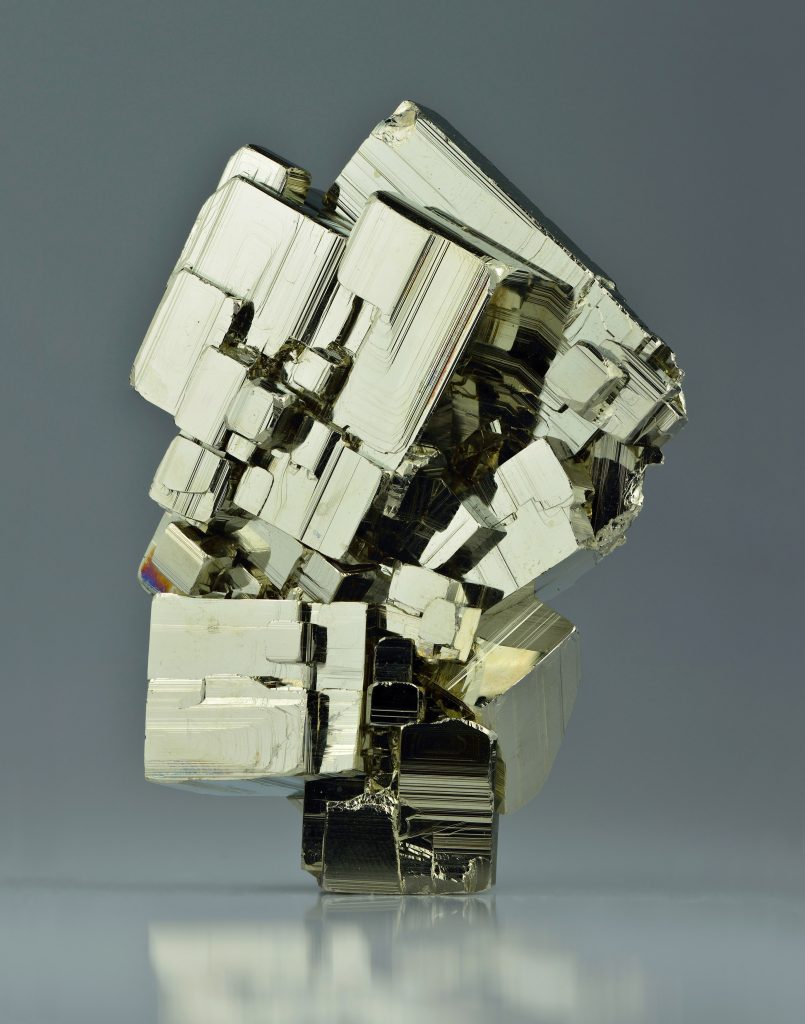
External crystal form
Most commonly, the term “crystal” describes a mineral that shows external flat surfaces determined by its internal crystal structure. In more advanced courses, you will learn to classify minerals into crystal systems, such as cubic, hexagonal, monoclinic, based on the symmetry of both the internal crystal structure and the external crystal form.
External crystal form is most commonly shown by minerals that have grown into a large fluid-filled space within the Earth, known as a geode.
Cleavage
In some crystals, atoms are only weakly bonded across certain surfaces that have a regular orientation in the crystal lattice. Such minerals are easily split along these flat surfaces. Such a property — breaking along flat surfaces controlled by crystal structure — is known as cleavage.
Calcite, the most common form of calcium carbonate CaCO3 shows three different orientations of cleavage, causing the crystals to break into rhombohedra — six-sided blocks each side of which is a parallelogram.
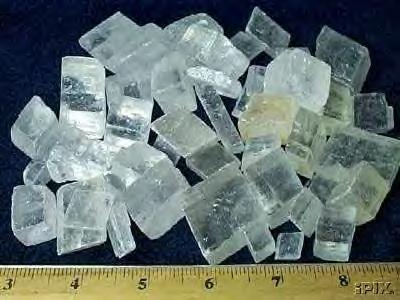
Fracture
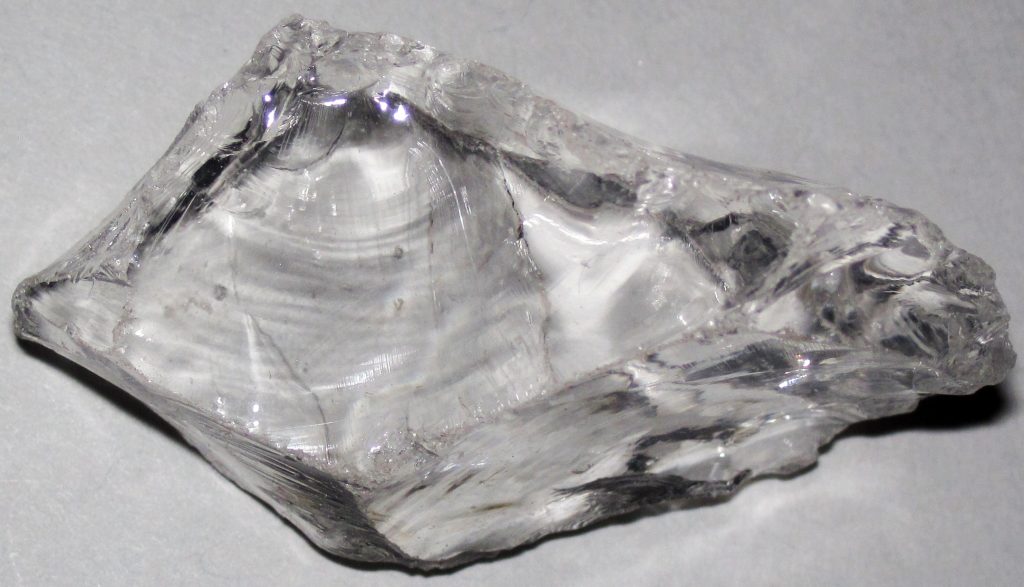
Minerals that don’t show cleavage break along irregular surfaces. This property is known a fracture. Although several types of fracture can be distinguished, we will mention only one here: conchoidalfracture describes a curving style of fracture with concentric lines shown by broken glass and the mineral quartz (SiO2). The curving surfaces can be likened to shell of a mollusc or chonch, giving rise to the name conchoidal.
Hardness
The overall strength of bonding between atoms in a mineral determines its hardness — the ease with which it can be scratched. Minerals can be tested by scratching one against the other, and against common materials of known hardness. The Mohs scale ranks common minerals in order of hardness from 1 (softest) to 10 (hardest).
-
Testing kit containing samples of all ten minerals on the Mohs hardness scale. By Hannes Grobe – Own work, CC BY 3.0, https://commons.wikimedia.org/w/index.php?curid=25055190 Talc
- Gypsum[6]
- Calcite
- Fluorite
- Apatite
- Feldspar
- Quartz
- Topaz
- Corundum
- Diamond
Some common materials can be placed on this scale: Fingernail 2.5; Copper coin[7] 3.5; Knife blade 5.5.
Therefore, if you can scratch a mineral with your fingernail, the hardness is below 2.5. Conversely, if a knife will not scratch the surface of the mineral, the hardness is above 5.5. When scratching minerals it’s important to try each object against the other. Sometimes a softer mineral will draw a line of powder on a harder mineral, mimicking a scratch!
Other useful properties for mineral identification
Density
Minerals vary greatly in density[8]. Carefully measured, density may be given in kg/m3 or in g/cm3 (or g/cc). For example quartz has a density of 2650 kg/m3 or 2.65 g/cm3, a pretty average value for rock-forming minerals. People who handle rocks habitually get used to this density; when they heft a sample containing (for example) hematite (7.9 g/cm3) or (if they are lucky) gold (19.3 g/cm3) they immediately notice the extra density. To make accurate determinations of density, it’s necessary to weigh a sample in and out of water, using Archimedes' principle.
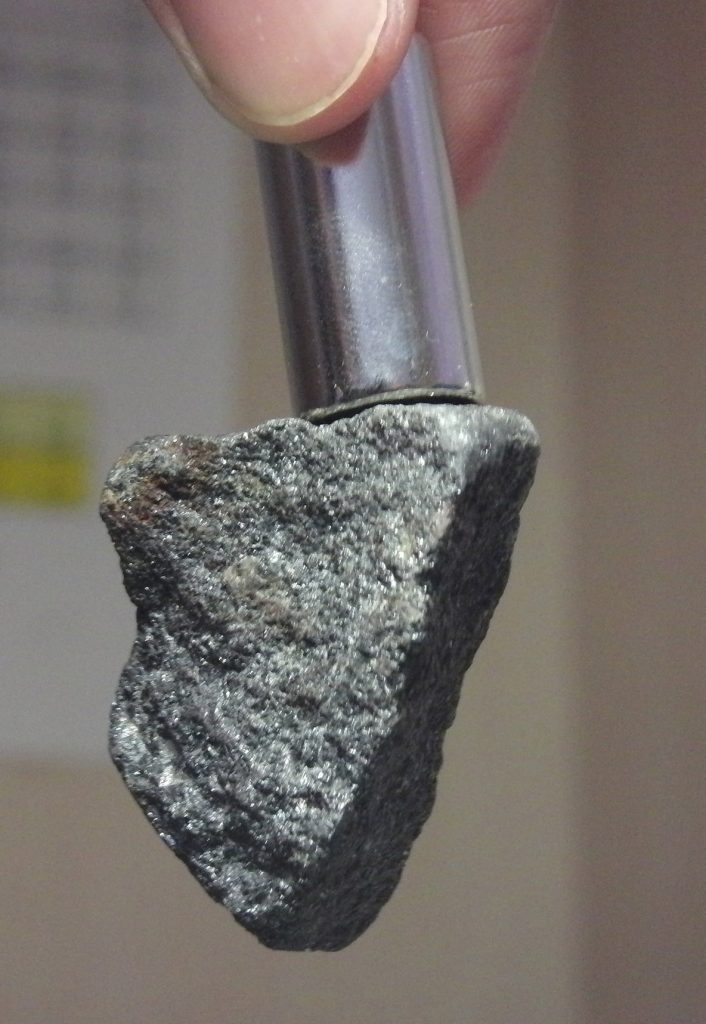
Magnetic properties
A few minerals are magnetic, in the sense that a magnet will stick to a sample of the mineral. The most important example is the iron oxide mineral magnetite Fe3O4. The magnetic properties of magnetite become important for the study of paleomagnetism, used to track the motion of plates in tectonics.
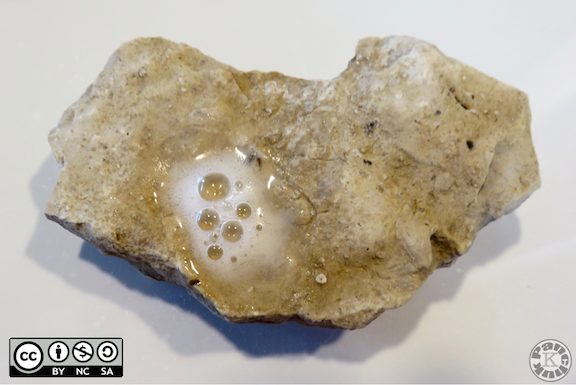
Reaction with acid
Carbonate minerals, including particularly calcite (CaCO3) effervesce (fizz) when a drop of dilute hydrochloric acid is applied to the surface. Geologists often carry a small bottle of dilute hydrochloric acid when working in the field, for the purpose of identifying carbonates.
Silicate minerals
Because oxygen is the most abundant element in the Earth’s crust, and silicon is the second most abundant element, it’s not surprising that the most abundant rock-forming minerals belong to a group called silicates, in which silicon and oxygen are combined with other metals in a variety of mineral structures.
Silicate tetrahedron
Silicate minerals have certain parts of their atomic structure in common. At the heart of all silicate mineral structures is a group of atoms called a a silica tetrahedron (silica is just a name for silicon oxide), a silicate tetrahedron, or an SiO4 tetrahedron. These three terms mean the same thing and can be used interchangeably. This structure arises because silicon, like carbon, has four electrons and four spaces in its outer electron shell, and silicon can form covalent bonds sharing one electron with each of with four oxygen atoms.
Each oxygen in the silica tetrahedron is left with one vacant space in the outer shell, and it can acquire electrons either in covalent bonds (often to other tetrahedra) or in ionic bonds to other metals, and we see both of those in silicate minerals.
In some minerals — typically those with lower amounts of silica compared with other metals — the SiO4 tetrahedra are isolated from one another, but in others they share oxygen atoms at their corners, building up strucutres in which tetrahedra are linked in rings, chains, sheets, or three-dimensional frameworks.
![]() |
![]() ![]() |
|
![]() |
![]() |
|
In general, the structures with more sharing of oxygen atoms between tetrahedra have smaller amounts of other elements (because there are fewer spaces left for electrons to be accepted by oxygen). The most extreme case is quartz, in which every oxygen is shared with an adjacent tetrahedron in a three-dimensional framework. The resulting formula is pure silica SiO2.
This simple picture is modified in some silicates, where aluminum (Al), with only 3 shareable electrons, sits in the middle of some of the tetrahedra. It is said to substitute for silicon. This frees up extra space for electrons in the outer shell of the oxygen atoms, which in turn allows ionic bonding with other metals. The feldspar group of minerals, the most abundant group in the Earth’s crust, has this type of structure, accommodating sodium, potassium, or calcium in a three-dimensional framework of SiO4 and AlO4 tetrahedra.
Minerals with isolated silicate tetrahedra
Our first mineral group is olivine, which is built from isolated silicate tetrahedra surrounded by ions of iron (Fe) and magnesium (Mg). This is a very important group because it consists of the four most abundant elements in the Earth, and especially in the Earth’s mantle. As you might expect this is most abundant mineral found in rocks from the mantle.
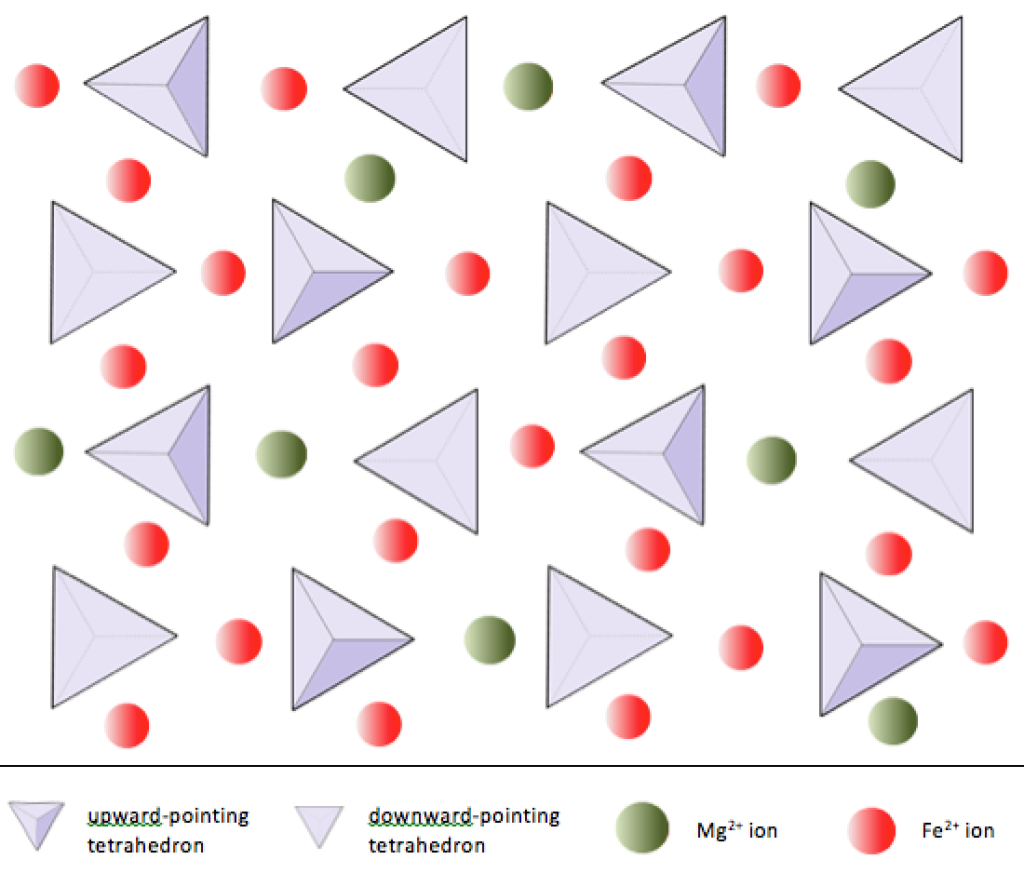
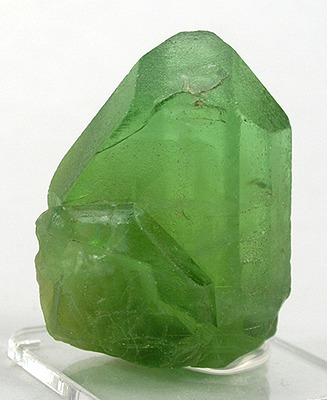
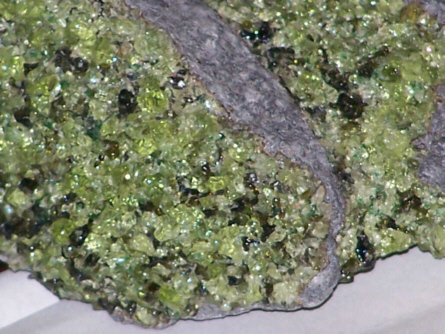
At greater depth…
It’s likely that olivine is not the most abundant in the deeper parts of the Earth’s mantle. Deep down in the mantle, the pressure is such that it can squeeze 6 oxygens around a silicon atom instead of four. In what’s called the mantle transition zone, from 450 to about 650 km deep, the common minerals from the Earth’s surface undergo phase changes to minerals in which the atoms are more closely packed together. One mineral believed to be common in the transition zone is ringwoodite, a polymorph of olivine. It is known from high-pressure meteorite impacts and was recently also discovered trapped inside a diamond that had been brought up by a volcanic eruption from deep in the mantle. Unlike olivine, it is bright blue, not green.
Below the transition zone, from 650 km to the surface of the Earth’s core, silicate minerals contain SiO6 octahedra, not SiO4 tetrahedra. A reddish brown magnesium silicate called bridgmanite, with SiO6 octahedra, is thought to predominate in the lower mantle, making it the most common mineral in the Earth, despite the fact that Geosphere bridgmanite has never been seen at the surface! However, bridgmanite has been recorded in meteorites, and bridgmanite has been synthesized in laboratory experiments that simulate lower-mantle conditions.
Olivine displays another important feature of silicate minerals. The ions of magnesium Mg2+ and Fe2+ have identical charge and are almost exactly the same size. They can easily substitute for one another, and in fact a continuous range of compositions is possible, from a pure magnesium end-member Mg2SiO4 to a pure iron end-member Fe2SiO4. This range is called a solid solution. It’s important to note that this variability affects only the iron and magnesium. The proportion of the total amount of Fe + Mg in the crystal is constant, so we can write a general formula:
(Mg,Fe)2SiO4
Most olivine in the Earth’s upper mantle contains much more magnesium than iron: about 90% of the crystal sites are occupied by magnesium and 10% are occupied by iron.
Solid solution between magnesium and iron is very common in silicate minerals. Because of the way light interacts with these ions, most such minerals have colours ranging from black to green. Olivine, as its name implies, is typically green. We refer to these minerals as ferromagnesian minerals.
Chain silicates
Minerals that contain larger amounts of silica typically contain oxygen atoms that are shared between adjacent tetrahedra. In simple chain silicates, each tetrahedron shares two oxygen atoms, leaving two to form ionic bonds with metals. The most important group is the pyroxene group, which forms the second most important component of the upper mantle.
Pyroxenes are also ferromagnesian minerals, and most have colours between green and black. A common example has the formula:
(Mg,Fe)SiO3
However, some pyroxenes contain calcium too, occupying exactly half of the available ionic sites in the crystal lattice. A typical formula is:
(Mg,Fe)CaSi2O6
The calcium ions have the same charge as the magnesium and iron ions but their size is different. They therefore fit in a different place in the crystal lattice, and the amount of calcium is fixed; it does not participate in a solid solution. Notice that to avoid fractions in the formula, it’s necessary to double the amount of Si and O in the formula but the ratio remains unchanged, so the overall structure is similar.
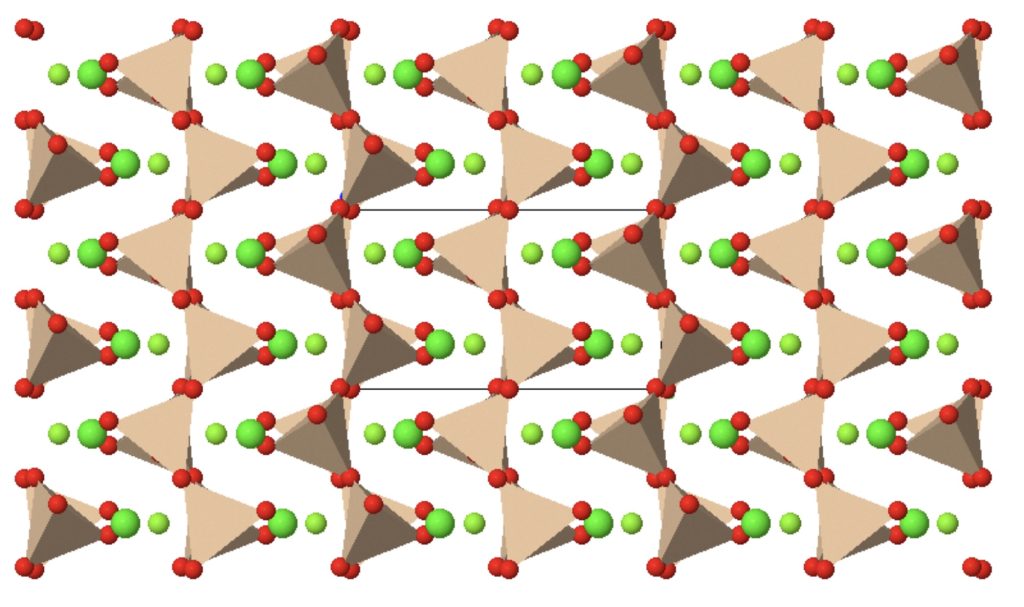
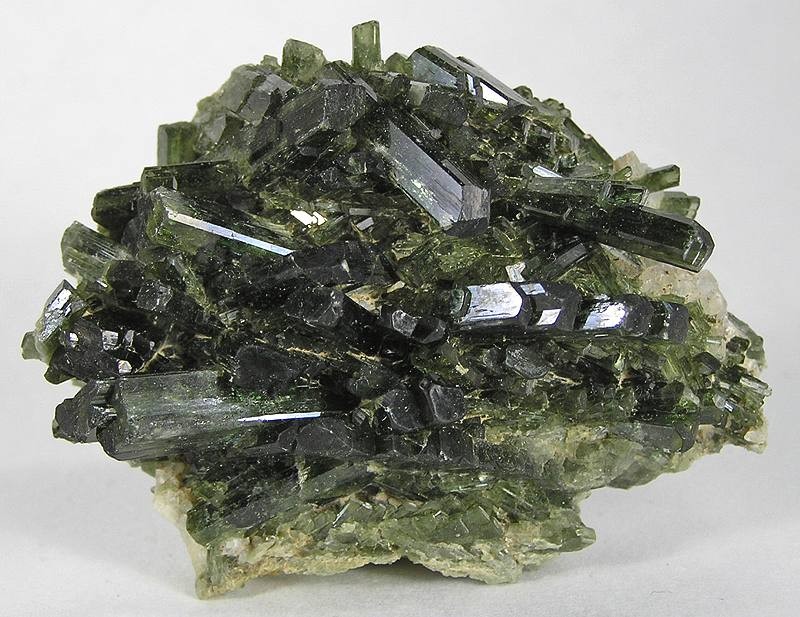
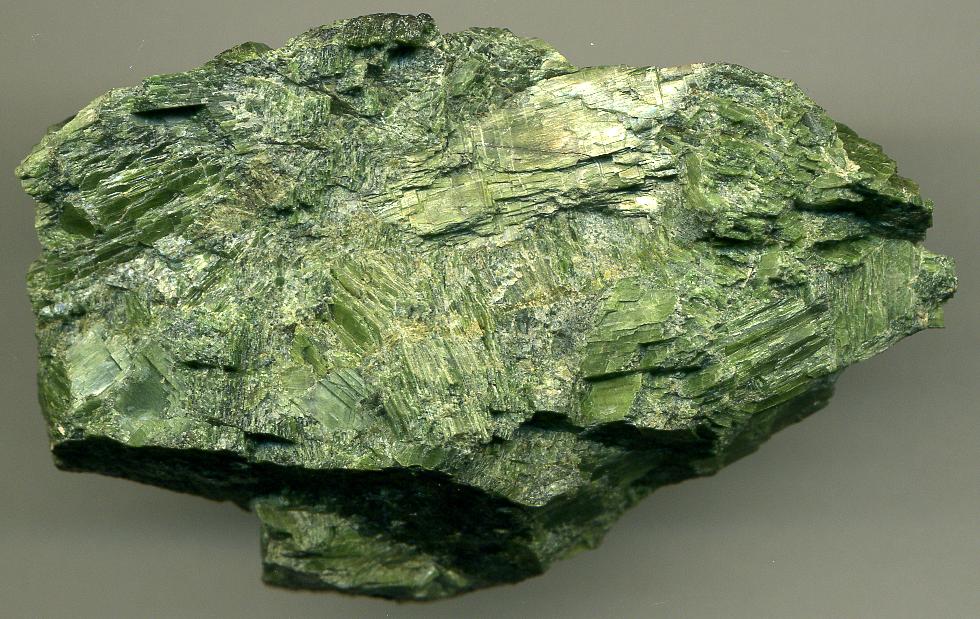
Pyroxenes typically form rather rectangular-shaped crustals with two sets of cleavage planes almost at 90° to one another. Pyroxene, together with feldspar, is a very common component of igneous rocks formed from magma that rises from the mantle.
Sheet silicates
Micas
If three of the oxygen atoms are shared between silica tetrahedra, the result is a continuous sheet, characteristic of sheet silicates. Micas are the best-known group. In most cases there is some aluminum subsituted for silicon in the sheets, and also some oxygen atoms are bonded to hydrogen in hydroxyl groups, and other elements like Fluorine may get involved. This makes for complicated formulas![10]
K(Mg,Fe)3AlSi3O10(F,OH)2
As you can see from the above formula, some micas are ferromagnesian minerals with solid solution of iron and magnesium. Such micas tend to be dark in colour — black or greenish like most ferromagnesian minerals.
Other micas, known as white mica, have aluminum in the equivalent position in the crystal lattice. White mica is not a ferro-magnesian mineral.
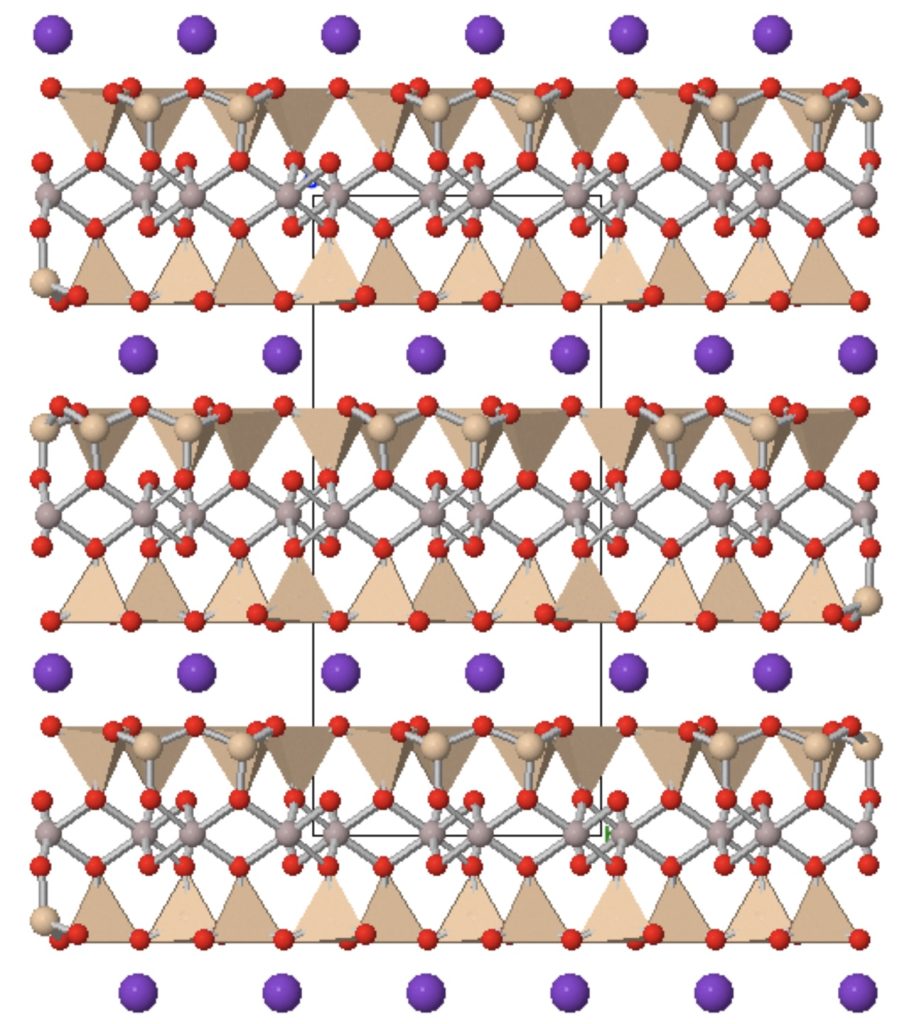
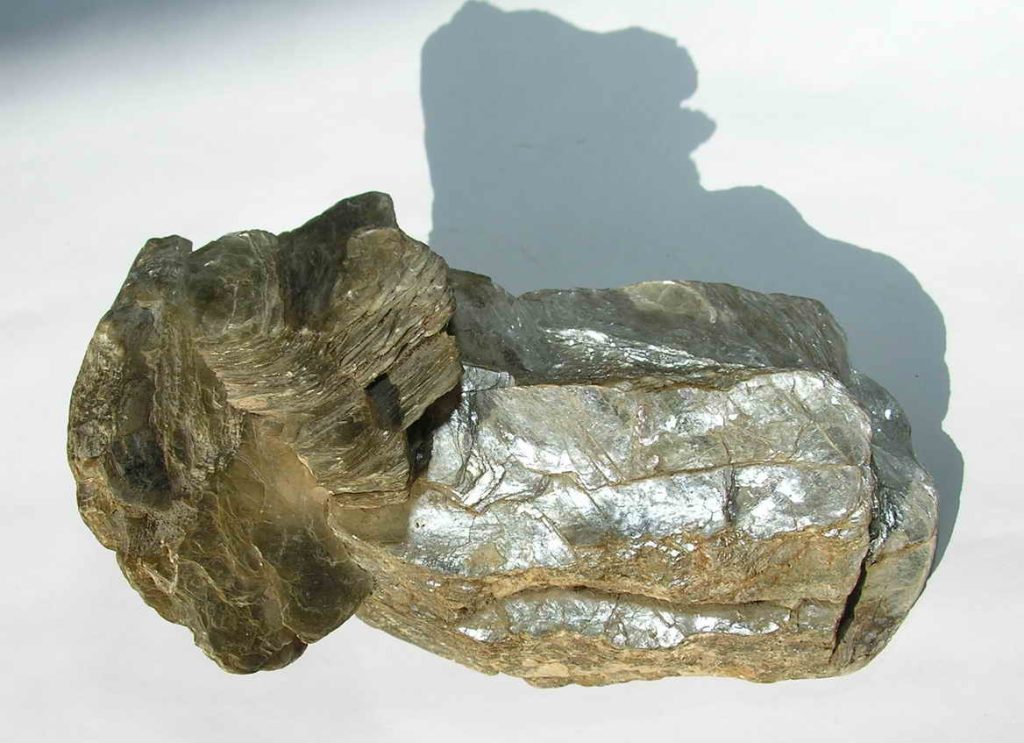
Whereas the silicate sheets are held together by strong covalent bonds, the sheets are ionically bonded together by potassium ions, and these bonds are much weaker. This allows mica to split into astonishingly thin, elastic sheets. Large crystals of white mica have historically been used instead of window glass. Mica is also used in electrical components and as a source of “glitter” for greeting cards.
Micas, both light and dark, are common components of metamorphic rocks formed by the action of heat, pressure, and deformation, particularly on sedimentary rocks. They are also an important component of silica-rich igneous rocks produced by melting in the crust. (Most magma formed by melting of the mantle does not usually contain enough aluminum to form mica. An exception is kimberlite, the igneous rock from which diamonds are obtained, which typically contains magnesium-rich mica).
Clay minerals
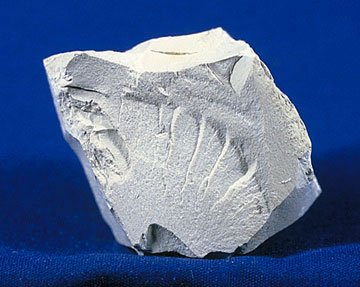
Sheet silicates are also produced in abundance by the processes of chemical weathering. The mineral grains produced tend to be very small — only a few microns across — making identification with the naked eye very difficult. (To identify them, a technique called X-ray diffraction is commonly used.) These fine-grained minerals, mostly sheet silicates, are collectively known as clay minerals. As the name implies, they are the main component of clay, used to make pottery of all kinds.
One example you may meet if you are taking a lab course is kaolinite, which has a relatively simple formula (for a sheet silicate):
Al2Si2O5(OH)4
You can see that it’s an aluminum silicate with included hydroxyl ions. Kolinite is formed by the weathering of feldspars under tropical conditions, and is the principal component of china clay used to make porcelain and fine china pottery.
Framework silicates:
Quartz
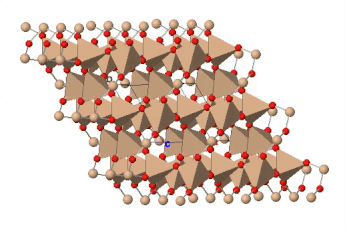
If all the oxygen atoms in silicate tetrahedra are shared, the resulting three-dimensional structure is entirely covalently bonded; there are no empty spaces available in the outer electron shells of the oxygen atoms for ionic bonding. The resulting structure has the formula SiO2 and represents the mineral quartz. Quartz is a common component of many kinds of rock: sedimentary, igneous and metamorphic. Quartz is the most abundant single mineral in the Earth’s continental crust, although the minerals of the feldspar group, taken as a whole, are more abundant. It is much less common in the oceanic crust, which contains less silica, and largely unknown in rocks derived from the mantle.
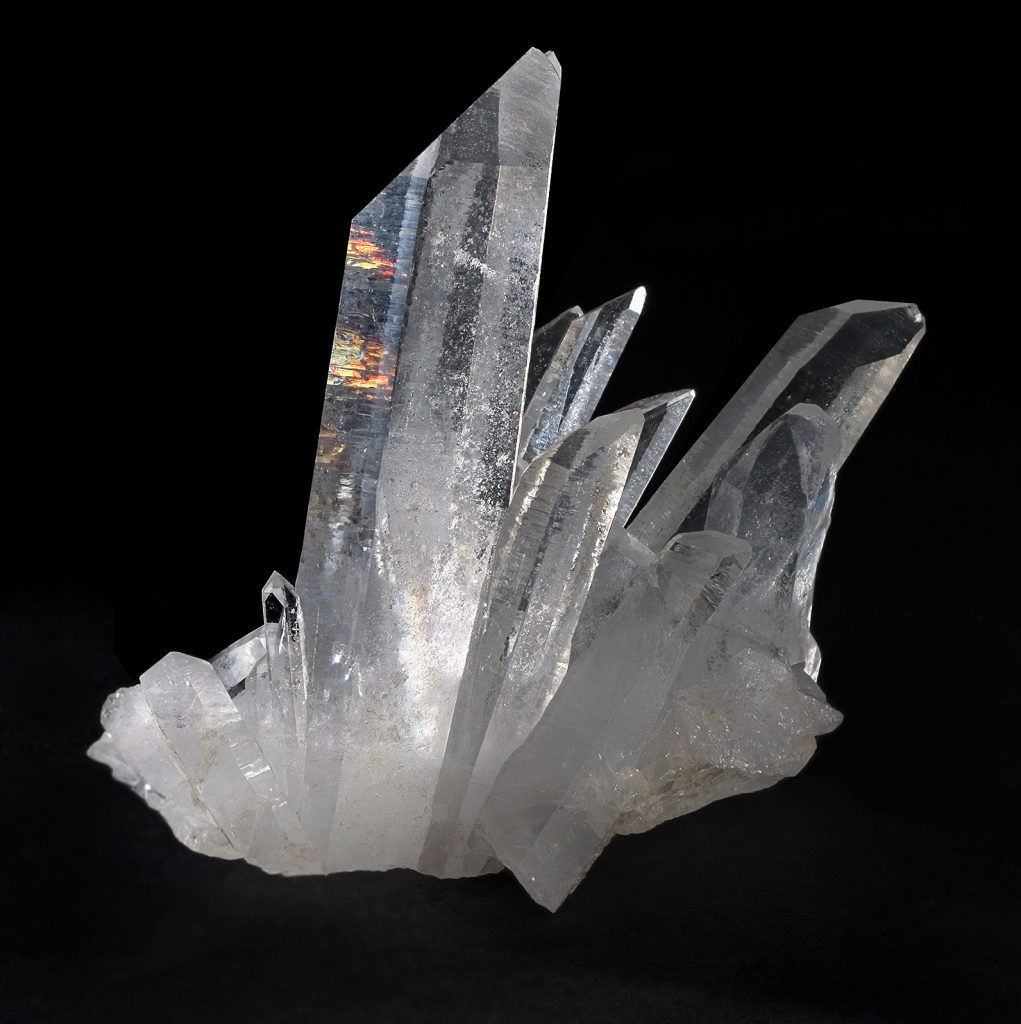
Quartz is recognizable by its hardness — it’s the hardest of the common rock-forming minerals — and lack of cleavage. Internally, the silicate tetrahedra are linked in a spiral arrangement without through-going planes of weakness. When broken, it displays conchoidal fracture. Colour is a poor guide to identification: quartz can occur in almost any colour. Some of those colours have particular names and are valued as semi-precious stones.
Feldspar group
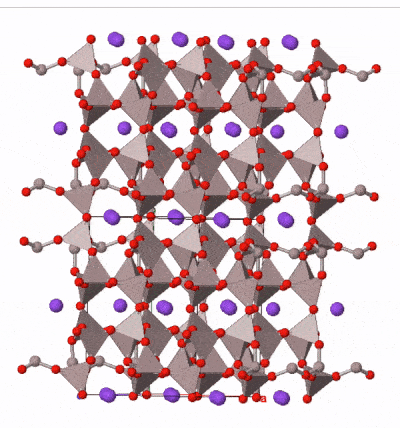
The most common silicate group of all is the feldspar group. Examples are found in almost all igneous rocks. Feldspars are framework silicates in which every oxygen atom is shared between two tetrahedra, but a proportion of the tetrahedra (between one quarter and a half) contain aluminum atoms instead of silicon, leaving space in the outer shell of some of the oxygen atoms for either one or two electrons. These electrons may be derived from ionically bonded sodium Na+, potassium K+, or calcium Ca2+. A variety of solid solutions are possible between end-member compositions:
KAlSi3O8
NaAlSi3O8
CaAl2Si2O8
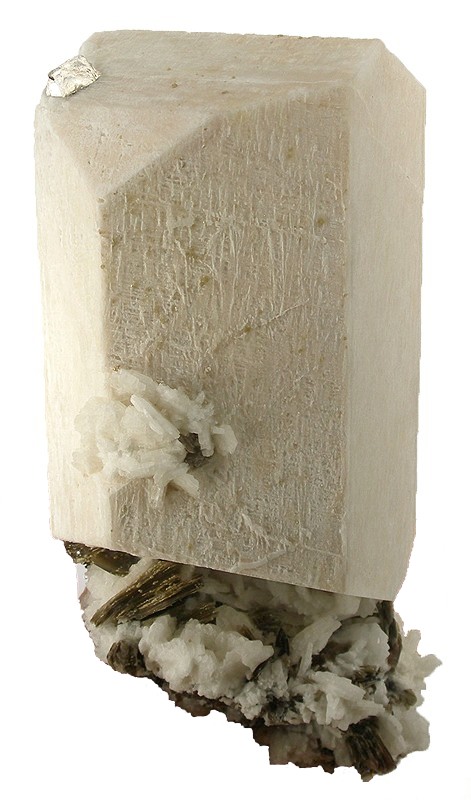
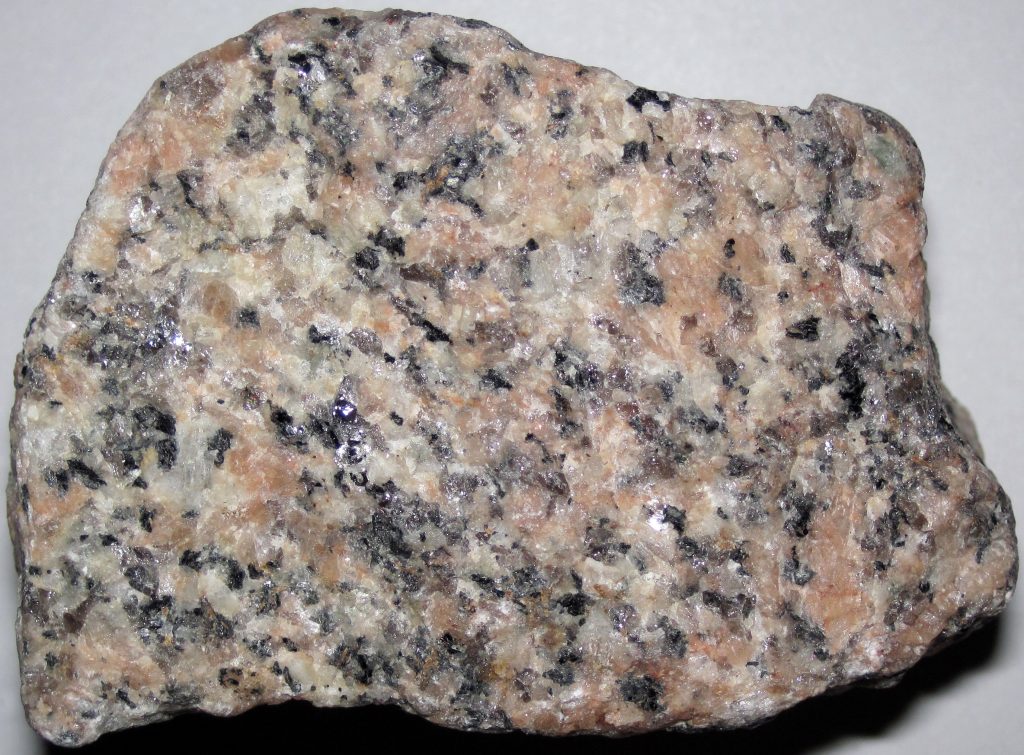
Feldspars form blocky, typically box-shaped crystals, with two cleavages approximately at right angles. Sometimes they contain twin planes across which the mineral structure is reflected into mirror images. These are sometimes visible when rotating a crystal in a beam of light. Colours range from pink (common in potassium feldspar) through white to medium grey (common in sodium and calcium feldspar) although more detailed study of optical properties under a microscope is necessary to securely determine the composition of a feldspar.
In detail: Labradorite
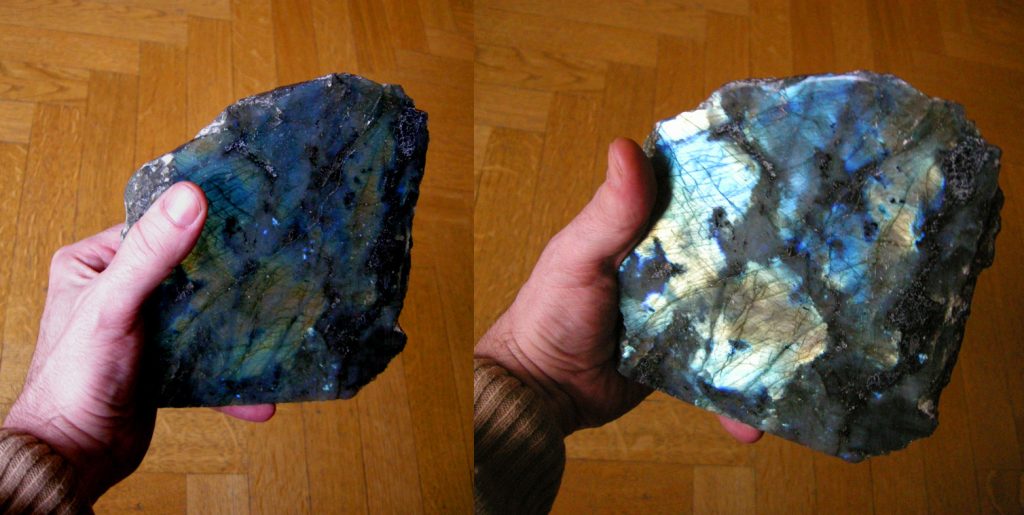
Solid solution occurs more readily in feldspar at high temperature. Almost any composition between the Na and Ca members is possible. However, as feldspars cool down, they tend to “unmix”, forming sub-microscopically thin plates of different composition. In the variety labradorite, containing roughly 60% calcium and 40% sodium, these thin plates are just the right thickness (comparable to the wavelength of visible light) to cause a play of colour called schiller, a distinctive and prized property of labradorite.
Taken as a whole, feldspars are the most abundant group of minerals in the Earth’s crust. The Earth’s mantle contains much lower concentrations of the elements in feldspar, and so feldspar is rare in the mantle. It occurs where the mantle is present at shallow depths beneath oceanic crust, but at depths greater than 10–15 km, aluminum is taken up by another mineral group, spinel, and below 50 km by garnet, so feldspar is not present in deeper parts of the mantle.
Non-silicate minerals
Non-silicate minerals make up only about 5% of the Earth’s crust. Why then do we devote a whole section of this chapter to them? The reason is that non-silicate minerals, ranging from common limestone to extremely rare gold and diamonds, have been sought after by humans for milennia. Many of the minerals we encounter in this section have important uses in human technology, and are therefore mined in different parts of the world.
Carbonates
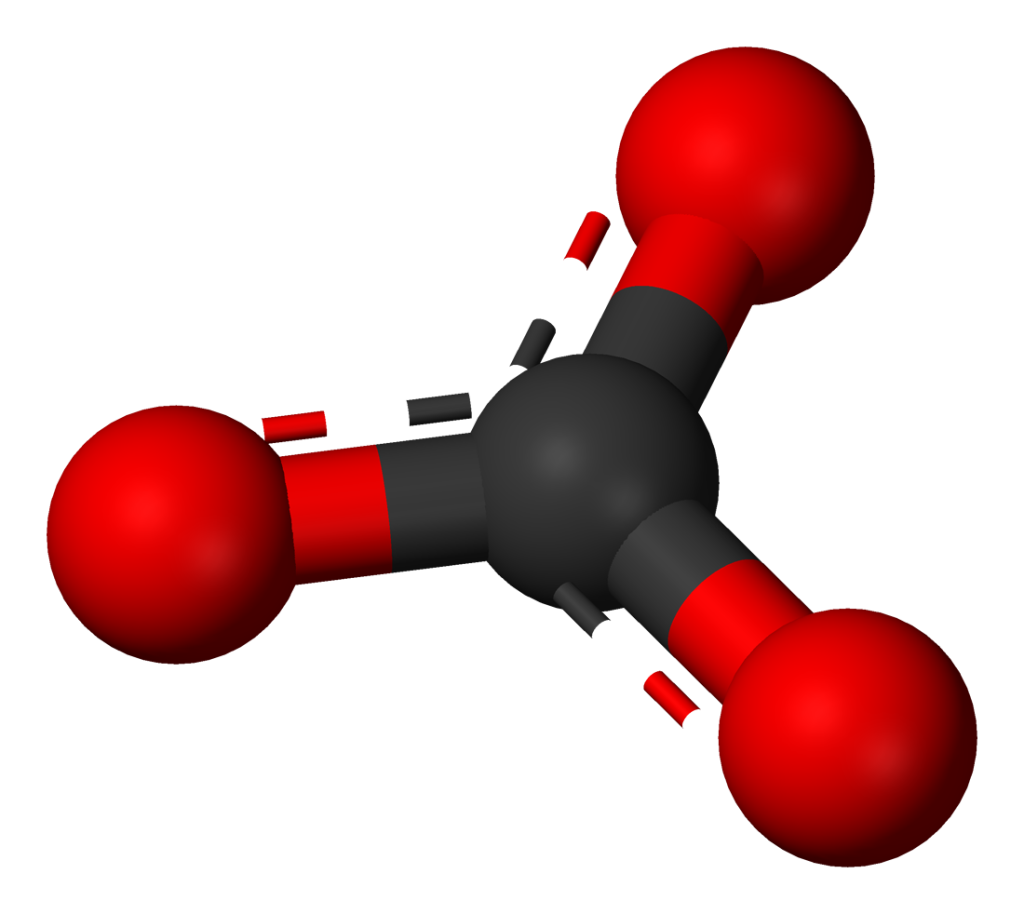
Carbonate ions figure prominently in the carbon cycle, because they are one of the forms of oxidized carbon that can occur in the Hydrosphere. Carbonate ions contain one carbon atom covalently bonded to three oxygen atoms; the overall ion carries a double negative charge.
CO32-
There are a number of important rock-forming minerals that are combinations of positive metallic ions and negative carbonate ions. The most important is calcite, with the formula CaCO3, in which Ca2+ ions are combined with carbonate ions in a structure that has three-fold rotational symmetry.
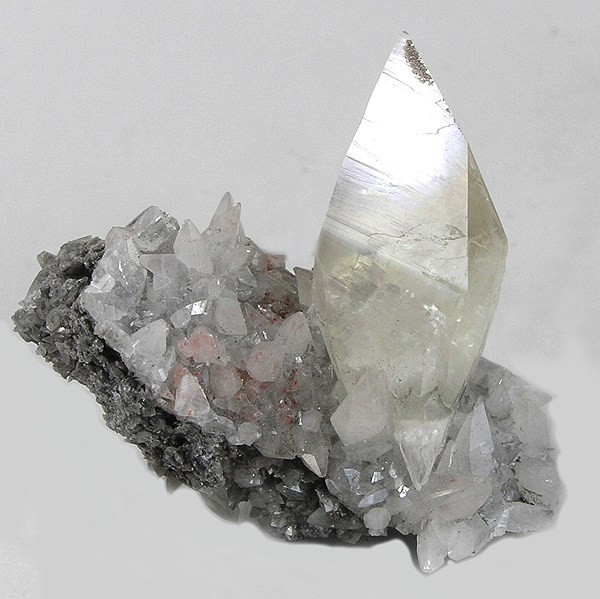
Calcite sometimes appears similar to quartz, but is much softer (hardness 3, so it can easily be scratched with a knife), and calcite effervesces when a drop of dilute hydrochloric acid is applied. Its cleavage is also distinctive, causing it to break into rhombohedral fragments.
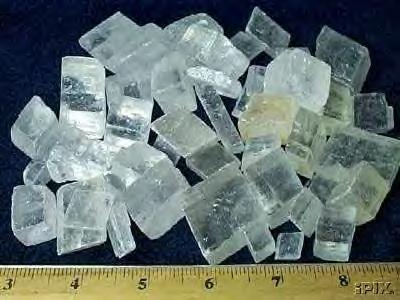
Calcite is formed by many organisms, including cyanobacteria, microscopic planktonic plants called coccolithophores, many corals, crustaceans, molluscs (clams and snails), and echinoderms (starfish and sea urchins). All these organisms build their shells or other skeletal material using calcium and carbonate ions extracted from water.
Calcite is the principal mineral component in the sedimentary rock limestone, and in its metamorphosed equivalent marble. Calcite is also deposited from groundwater, forming stalagmites and stalactites of dripstone in caves, and calcite veins that fill fractures in the Earth’s crust. Calcite, in the form of limestone, is mined in large quantities for the production of cement, and in smaller amounts for agricultural lime, used to increase the pH of acid soils.
Halides
A second group of minerals consist of ionically bonded combinations of metals that have one free electron in the outer electron shell (alkali metals from Group I of the periodic table) with halogens from group VII— elements with one free space for an electron in the outer shell. We have already met the most common mineral in this group: halite or common salt, sodium chloride NaCl.
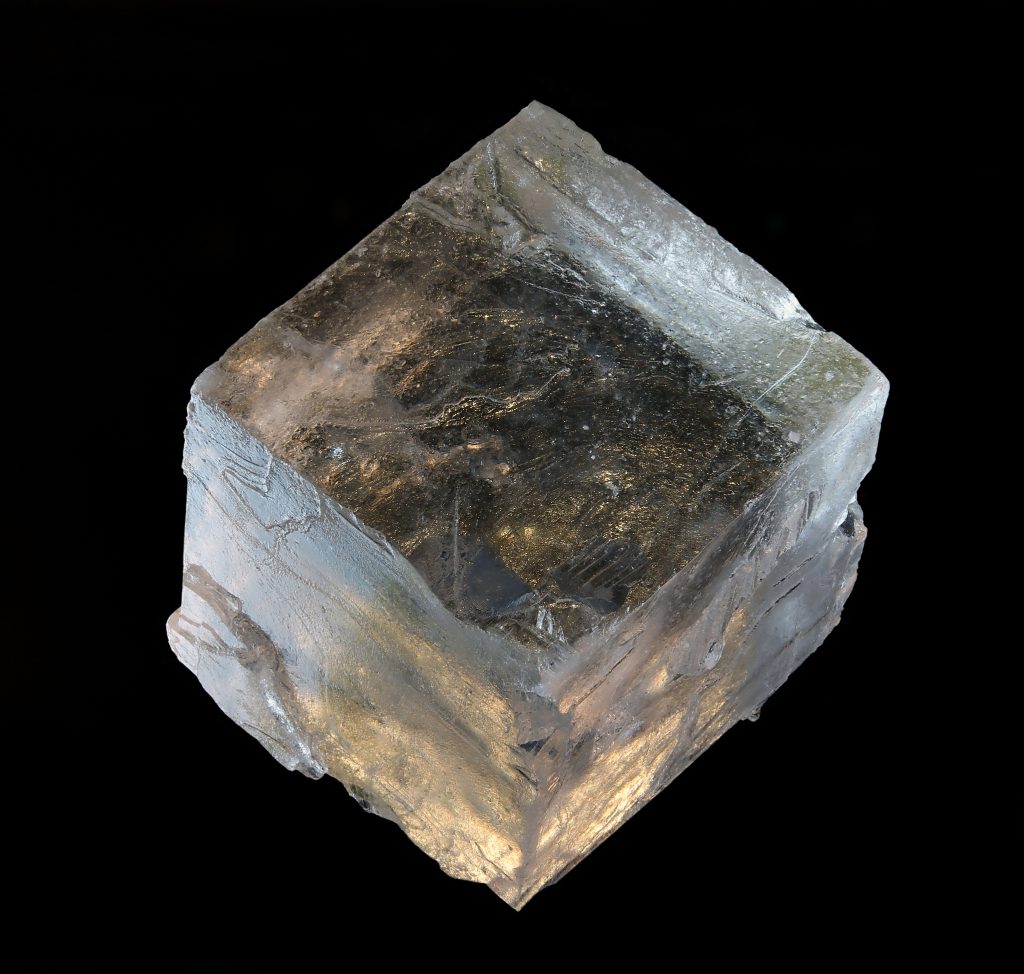
Halite is a soft mineral (hardness 2, so it can be scratched with a fingernail) that crystallizes in perfect cubes, with cleavage planes parallel to the faces. Halite dissolves in water readily, but does not fizz with hydrochloric acid. When pure it is white or transparent, but it is easily coloured by traces of impurities.
Halite is typically formed by the natural evaporation of sea-water, and is therefore one of a class of minerals known as evaporite minerals. A rock formed mainly of halite is known as rock salt.
Sulfates
In addition to chloride ions, sea-water contains abundant sulfate[11] ions consisting of a sulfur atom oxidized by four oxygen atoms, carrying a net double negative charge. As a result, some sulfate minerals are also produced when sea-water is evaporated, and are included in the category of evaporites.
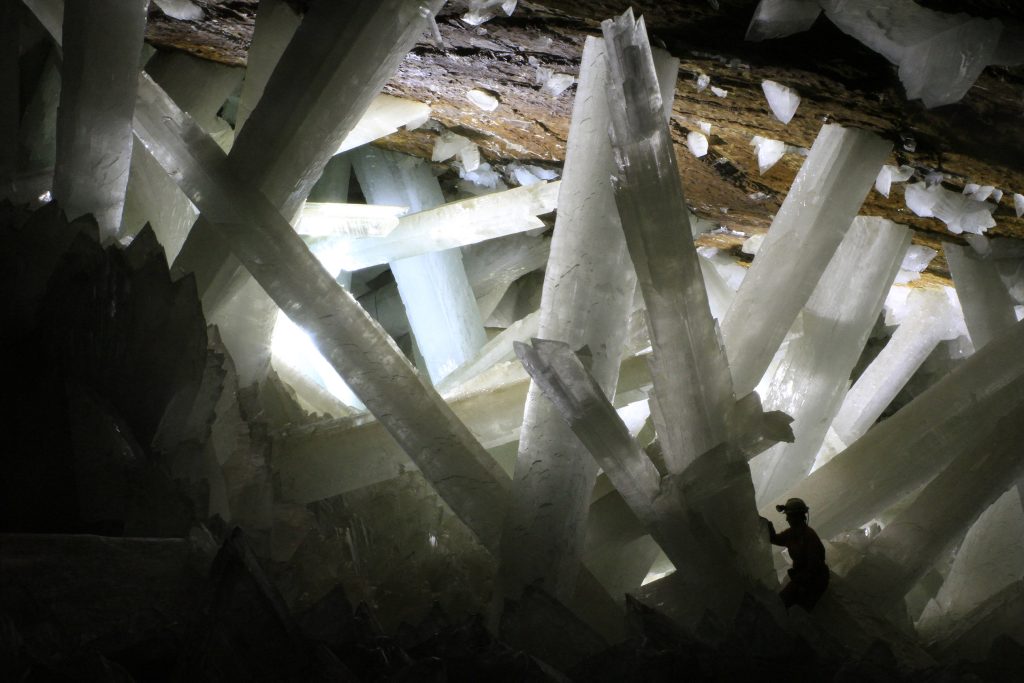
The most common is gypsum, hydrated calcium sulfate, which actually includes water molecules in its crystal lattice. Its formula is
CaSO4.2H2O
Gypsum is also soft (hardness 2) and crystallizes in a variety of forms including transparent diamond-shaped crystals called selenite, which displays perfect cleavage parallel to the large flat surfaces, and long thin fibres known as satin spar.
Gypsum is used by humans to make plaster. Heating gypsum drives off three quarters of the water, leaving a powder (Plaster of Paris) which turns solid when water is added. Plaster wall-board (“Gyp-rock”) is an important component of new homes in many parts of the world.
Sulfides
Not to be confused with sulfates are sulfides, minerals containing negative ions of reduced sulfur. Two of these are quite common, though many more occur as ores of various metals.
Pyrite
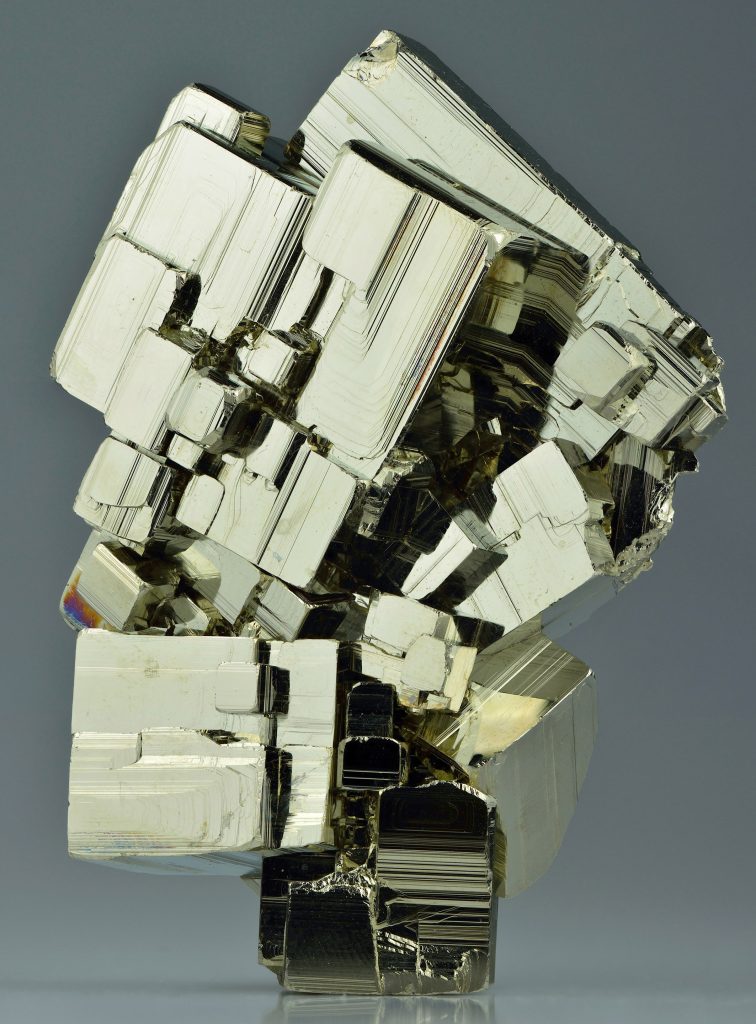
Pyrite FeS2 is a common sulfide mineral, containing Fe2+ ions ionically bonded to ions consisting of two sulfur atoms covalently bonded together and carrying a 2- charge. It’s common in organic-rich sedimentary rocks, where sulfate ions from sea-water are reduced after deposition (during a stage in sedimentary rock evolution called diagenesis) by organic carbon also present in the sediment.
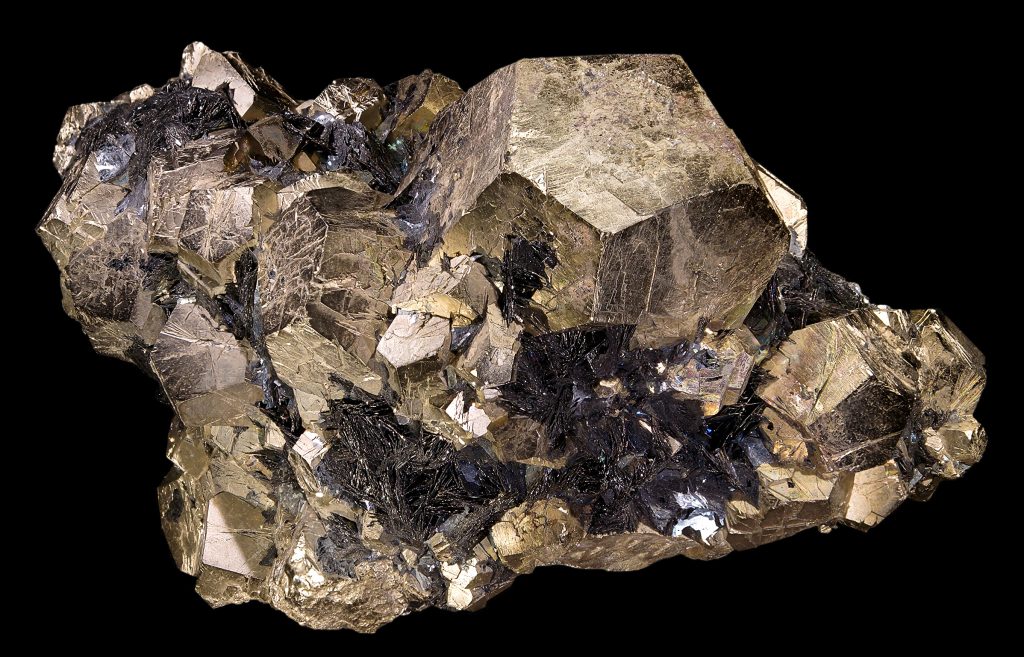
Pyrite has a distinctive yellow colour and metallic lustre. It is sometimes known as fool’s gold, but its hardness is 6–6.5, much harder than gold. Well formed crystals are commonly cubic, but sometimes form dodecahedra with twelve pentagonal faces. Pyrite shows no cleavage, breaking instead along irregular rough surfaces.
Pyrite is not a commercial ore of iron, because removing the sulfur is a difficult and environmentally damaging process compared with extrating iron from its oxides.
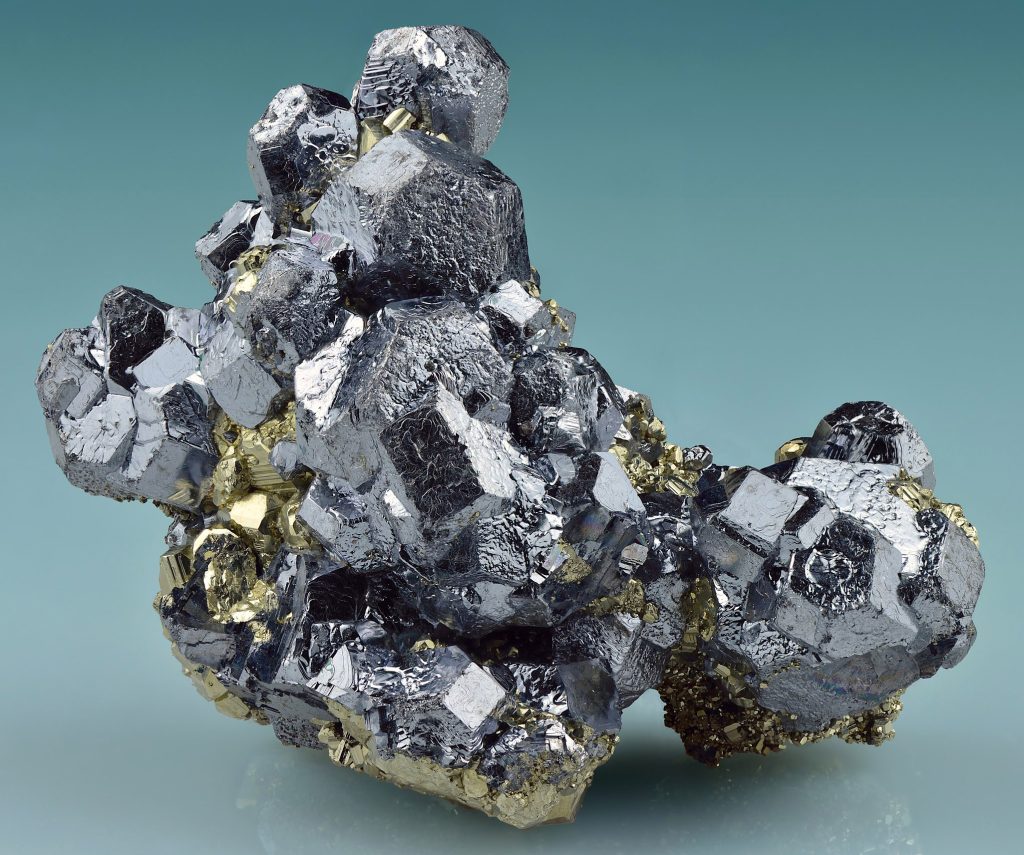
Galena
A second sulfide mineral is lead sulfide galena with the formula PbS2. Galena also crystallizes in cubes (though not dodecahedra), but its hardness is about 2.5 (much softer than pyrite) and it’s grey and metallic in colour and lustre. Galena shows perfect cubic cleavage, breaking into small cubes when struck with a hammer.
Galena is an important ore of lead, occuring in vein systems particularly in carbonate rocks. In some cases, galena contains silver as an impurity; the silver may be more valuable than the lead when galena is mined.
Oxides
Oxides are also an important class of minerals, forming ores of several metals that are mined by humans. We will look at two, both ores of iron. Iron can exist in two oxidation states. In iron II, a reduced form, two electrons are lost to produce Fe2+ ions as are common in ferro-magnesian minerals. However, oxygen, when present, can remove an extra electron, forming iron III, the oxidized form, which produces Fe3+ ions. Minerals containing iron III are characteristically red or orange in colour, in contrast with the green to black colours characteristic of iron II compounds.
Magnetite
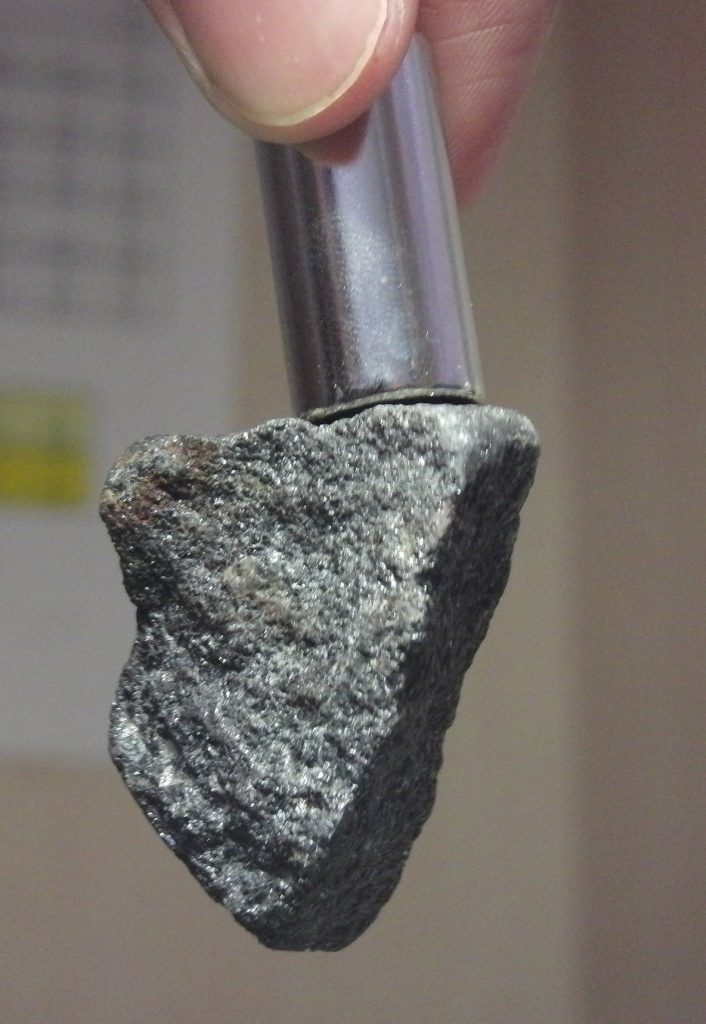
Magnetite contains a mixture of iron II and iron III producing the overall formula Fe3O4. Magnetite is a hard (hardness ~6) black mineral with metallic lustre. It is sometimes found in cubic crystals that lack cleavage. Its most distinctive property is that it is attracted to a magnet, and some magnetite (lodestone) is naturally magnetic. Magnetite is a major component of banded iron formations which are mined for the production of iron and steel. The extraction of pure iron from magnetite is carried out by reacting it with carbon (typically in the form of coal) producing iron or steel and carbon dioxide as a byproduct.
Hematite
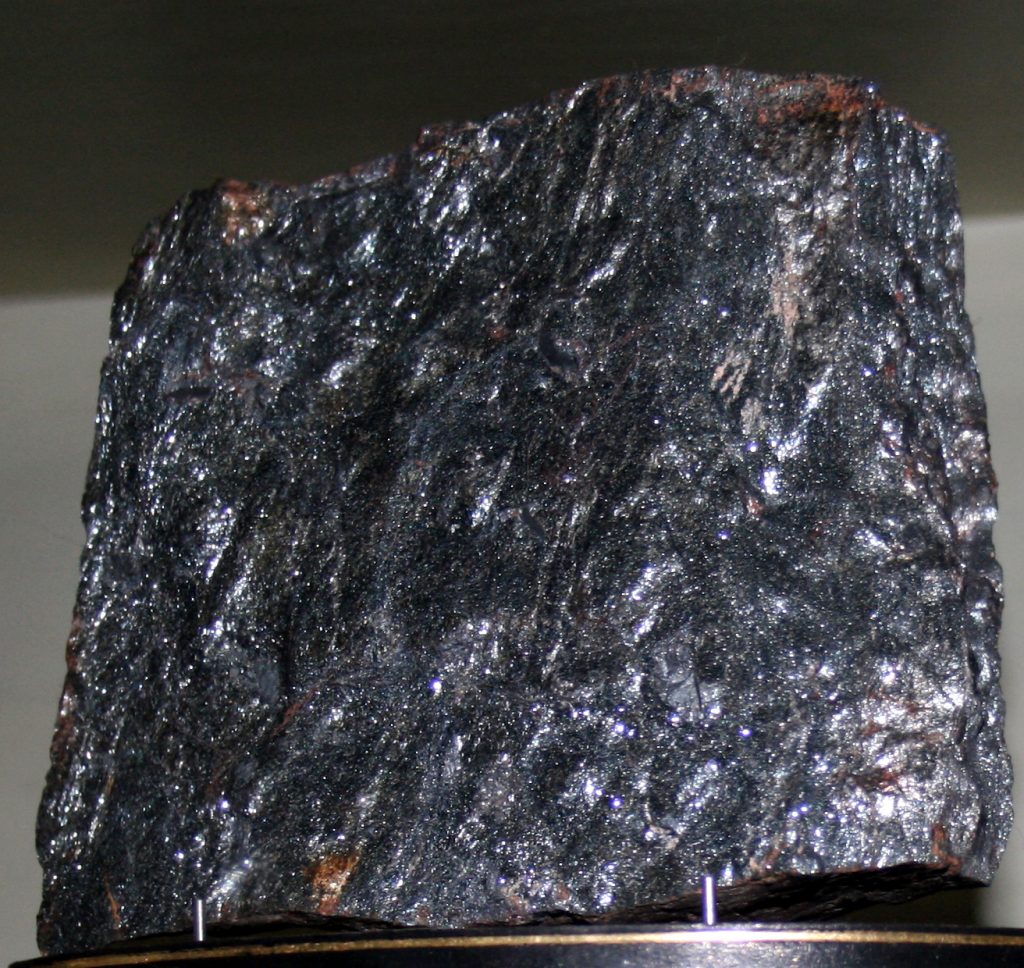
Hematite[12] is a purely iron III oxide Fe2O3. It may also be black and metallic (specular hematite) but is distinguished from magnetite by its lack of magnetism and its red streak. In fine-grained form it is an earthy red colour and has dull or earthy lustre. Very small amounts of hematite occur in many red sedimentary rocks, where it’s an indicator that the rock was oxidized at some point in its history. However, red colour does not indicate an abundance of iron; the colour of fine-grained hematite is so intense that only a tiny amount is required to make a very red rock.
Like magnetite, hematite is a major component of banded iron formations from which iron is mined in many parts of the world.
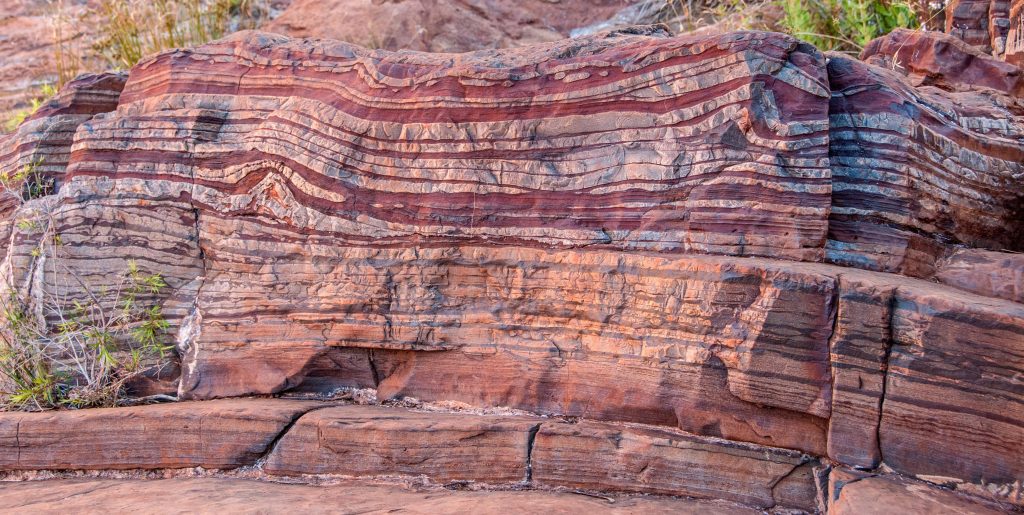
Native elements
Minerals are described as native elements when they consist one kind of atom, bonded only to other atoms of the same element. Only some elements in the periodic table occur naturally in this way. Two are particularly valued by humans for their appearance and durability.
Gold
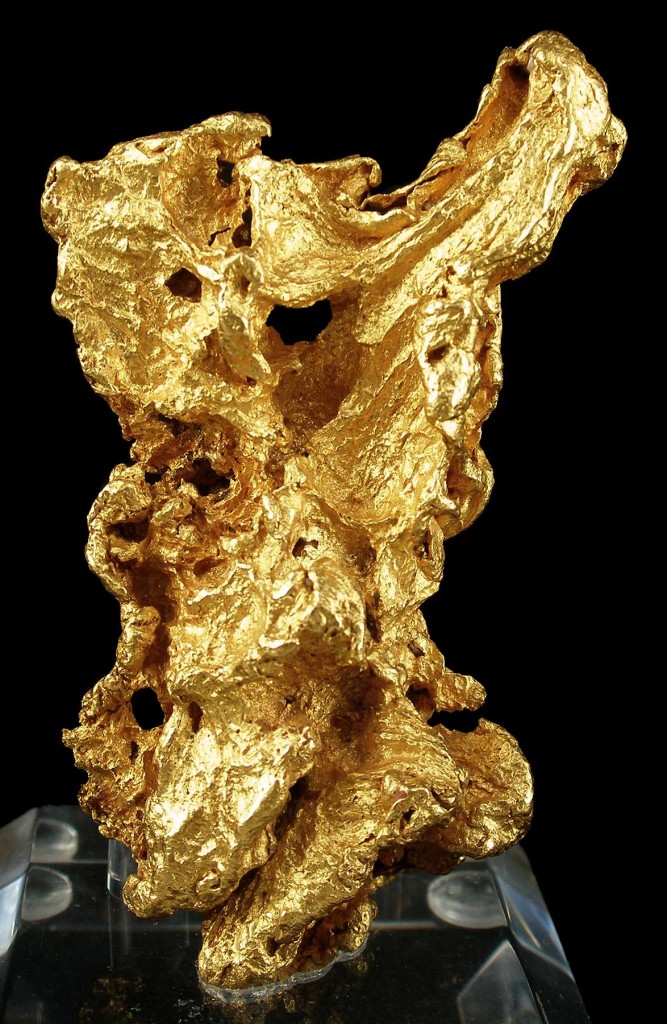
Gold (Au) is one of the densest minerals known, at 19300 kg/m3 or 19.3 g/cm3.[13]. It is over seven times denser than common rock-forming minerals like quartz, feldspar, and calcite.
Gold shows metallic bonding and is distinguished, if you are lucky enough to find it, by its softness (hardness 2.5) and the ease with which it can be worked into shapes with hand tools. Gold is principally found in veins where it was deposited, often with other minerals, from hot water flowing through cracks within the crust. However, it’s chemically very unreactive, and so tends to survive weathering and erosion of the rocks around it. Because of its density, it tends to be transported differently from more common minerals in streams, and gold particles may be concentrated in certain places, known as placer deposits. The density of gold enables other minerals to be separated from it by moving water when panning for gold.
Diamond
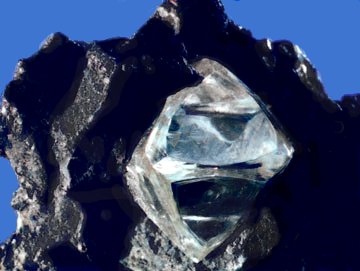
Diamond (C) is entirely covalently bonded, and as a result is the hardest mineral known. Diamond is a high-pressure polymorph of carbon and is only stable at great depth within the mantle. As far as we know, with the exception of small diamonds formed in meteorite impacts, all natural diamonds formed over 100 km down in the mantle where pressures are sufficient to stabilize diamond. Diamonds reach the surface through the eruption of kimberlite magmas in explosive eruptions that occur very rarely – no kimberlite eruptions have been recorded in human history, but many examples are known from the geological record.
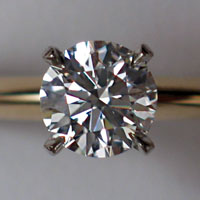
Diamond typically forms octahedral crystals. For jewelry, these are cut so as to maximize the play of colour. The optical properties of diamond cause different colours of light to be refracted differently as they pass through diamonds, giving it a special type of lustre called adamantine.
- Lead is a confusing word for non-English speakers (and even some who have spoken English for years). It's pronounced "led". Confusion arises because the word "led" itself is the past tense of the verb to "lead", confusingly spelled the same way as the element Pb but pronounced with a long 'ee' to rhyme with seed. ↵
- Aluminium in British English ↵
- The term allotrope is also used to describe a polymorph of a single element. However, it's simpler to use a single term for both elements and compounds that form more than one solid phase. ↵
-
↵
In greater depth
<In a future version we may include a phase diagram for andalusite, kyanite, and sillimanite here so as to include a phase diagram that is more useful in the section on metamorphic rocks> - Color in the United States ↵
- Bold names are important minerals that we meet elsewhere in this book ↵
- Since the demise of the Canadian 1¢ coin, or penny, copper coins have been hard to find. We keep a supply of old pennies for testing in our lab kits for our course in Edmonton. ↵
- Mineralogy texts often describe a property specific gravity, which is density compared to a reference material, usually water. Because water has a density of 1.0 g/cm3, the specific gravity is numerically the same as density in these units, but is technically a dimensionless number. ↵
- Chris Schaller. CC BY-NC https://chem.libretexts.org/Bookshelves/General_Chemistry/Book%3A_Structure_and_Reactivity_in_Organic_Biological_and_Inorganic_Chemistry_%28Schaller%29/I%3A__Chemical_Structure_and_Properties/10%3A_Network_Solids/10.03%3A_Silicates_and_Silica ↵
- In the introductory Earth science course for which this text is designed, it is not required or even recommended to learn complex formulas by heart! It's much more important to know what the formula represents and to be able to recognize components like solid solution series ↵
- The spellings "sulphate" and "sulphur" are often used in British English, though the spellings "sulfur" and "sulfate" are increasingly common. ↵
- sometimes "haematite" in British English ↵
- Osmium, another exceedingly rare native element, is denser, at 22 g/cc. ↵
The solid Earth
A visual representation of the known elements, by order of their atomic number. Columns represent elements having similar outer-shell electron configurations, and hence similar chemical properties
The central part of the Earth below about 2900 km from the surface, composed largely of metallic iron
The layer within the Earth or other inner planet that overlies the core and makes up the majority of the planet, composed chiefly of magnesium- and iron-rich silicates
Naturally occurring crystalline solids whose composition includes groups of silicon and oxygen atoms, typically in a covalently bonded tetrahedral arrangement
A thin, surface layer of the Earth that lies above the Moho; differentiated into thicker, lighter continental crust and thinner, denser oceanic crust
A type of meteorite characterized by small, roughly spherical particles of glass or former glass, known as chondrules
A naturally occurring crystalline solid with a fixed or limited range of compositions
A naturally occurring mixture of minerals, and in some cases other solid materials, that occurs in the Geosphere
The regularly repeating three-dimensional arrangement of atoms and/or ions in a crystalline solid material such as a mineral
Water in its solid, crystalline form
Carbon-rich sedimentary rock formed from solidified organic material that originated as peat
An abundant group of framework silicates containing aluminum together with some combination of
potassium, sodium and calcium
Silica SiO₂ in its most common mineral form.
A group of sheet-silicate minerals with a sheet-like habit and perfect cleavage.
Matter containing reduced carbon that originated in a living organism.
Igneous rock formed by rapid cooling of lava, such that crystal lattices are unable to form; rock with the structure of a supercooled liquid.
Bonds between atoms formed by the transfer of electrons from one atom to another. The resulting negatively and positively charged ions are attracted together.
An atom or group of atoms that has lost or gained one or more electrons, resulting in a net positive or negative electric charge
Positively charged ion
A negatively charged ion, that has gained one or more electrons relative to its constituent atom(s)
The form of bonding in metals, whereby a cloud of free electrons is held by a lattice of metal ions.
Atomic bonds wherein two atoms share electrons so as to acquire full outer electron shells.
Silicate Tetrahedron (Also silica tetrahedron or SiO₄ tetrahedron; plural tetrahedra): A group of four oxygen atoms covalently bonded to a silicon atom; the basic building block of silicate minerals.
A crystalline mineral form of elemental carbon the hardest known mineral.
A soft form of mineral carbon containing sheets of hexagonally linked carbon atoms weakly bound together
Minerals with the same chemical composition, yet a different structure.
A graphical representation showing the conditions of temperature, pressure, or composition in which different phases are stable
The part of a system that is separated from other parts by distinct boundary surfaces; for example water exists in three phases: solid ice, liquid, and vapour, which do not mix.
A state wherein a system is not in equilibrium, but an input of energy would be required to move it to an equilibrium state.
The sizes, shapes, and relationships between the mineral grains that make up a rock.
Lustre (USA: luster): The quality of light reflected from a mineral’s surface.
Vitreaous (glassy) lustre: Describes the image-forming reflection of light from the surfaces of a mineral that also has some transparency.
Relating to the properties of metals, substances that tend to conduct electricity and to form bonds by losing electrons
A mineral sulfide of iron FeS₂ that has yellow colour and metallic lustre.
Lead sulphide (PbS), a mineral displaying a cubic habit and metallic lustre.
The lustre of a granular mineral whose surfaces reflect light poorly. Similar to dull
The lustre of a mineral whose surfaces reflect light poorly. Similar to earthy
; A mineral form of oxidised iron (Fe₂O₃)
An unglazed tile used to obtain a streak colour by abrading the mineral against its surface
The colour of mineral when finely powdered
.
The study of minerals under the microscope in order to identify them.
Double refraction; the splitting of light into two polarised beams refracted in slightly different directions, yielding a double image
A category of crystal lattice distinguished by its symmetry
A pore space partially filled by minerals.
Cleavage (mineral): The behaviour of a mineral that splits along flat surfaces controlled by its crystalline structure
A solid shape the faces of which are all parallelograms.
Any surface across which a rock or mineral loses its strength, that is not controlled by the mineral structure
A curved or ribbed shape, seen in the fracture surfaces of many minerals and a few rocks such as chert and volcanic glass
A mineral form of hydrated calcium sulphate (CaSO₄·2H₂O)
CaCO3; the most common mineral form of calcium carbonate
Mass per unit of volume of a substance.
A dense yellow metal element (Au), atomic number 79.
When a body is partially or completely immersed in a fluid, it experiences an upthrust equal to the weight of fluid displaced.
An iron oxide mineral with the formula Fe₃O₄
The study of preserved magnetism in ancient rocks
Substances with silicon–oxygen groups in their atomic structure
An element or ion in a crystal lattice that replaces another, typically of similar size and electron configuration
A silicate of iron and/or magnesium with separate silicate tetrahedra in its structure; (Mg,Fe)₂SiO₄.
A mineral or mineral group in which two (or more) different elements may be present in a continuous range of proportions.
Silicate minerals that contain large amounts of magnesium and/or iron, typically in solid solution, and a relatively low amount of silica.
A group of mainly ferromagnesian single-chain silicate minerals common in mafic rocks
Molten rock
Silicates whose crystal structure contains sheets in which three oxygen atoms are shared between silicate tetrahedra.
Ultramafic tuff produced by explosive volcanic eruptions sourced from the mantle; the main source of diamonds.
Changes in the mineral composition of rocks as they are exposed to air and water at the Earth's surface, producing new minerals and dissolved material
Clay mineral: A fine-grained hydrous mineral; clay minerals are mainly sheet silicates, most characteristically
formed by chemical weathering
Clay (sediment or rock) A sediment or soft rock composed largely of clay minerals finer than 4 μm, used in the manufacture of pottery and porcelain.
A clay mineral formed of hydrous aluminium silicate. AlSi₂O₅(OH)₄
Clay almost exclusively composed of the mineral kaolinite; used in the manufacture of porcelain
A plane within a crystal across which the crystal lattice adopts a reflected or rotated orientation
A mineral oxide of magnesium and aluminum; also, a mineral group of similar structure in which different metallic ions are present (eg Fe, Mn, Cr).
A group of hard silicate minerals with isolated silicate tetrahedra containing combinations of aluminum, iron, magnesium, calcium and other elements. Garnets are most common in metamorphic rocks
Single-celled prokaryotes capable of photosynthesis and the release of oxygen. Cyanobacteria were responsible for the original oxygenation of the Earth’s atmosphere.
Unicellular phytoplankton that secretes plates (coccoliths) of calcium carbonate to cover their bodies. Chalk is formed from these plates.
Sedentary suspension feeders, closely related to sea anemones and jellyfish, which may build exoskeletons of calcium carbonate. They are major reef-builders in Earth’s oceans.
A large group of Arthropoda with calcite-reinforced exoskeletons
A phylum of invertebrates which typically produce shells composed of calcium carbonate; Mollusca include snails, clams, and octopus (a group that has secondarily lost the shell)
Sedimentary rock composed mainly of calcium carbonate
The metamorphosed product of limestone. Marble contains coarse, interlocking calcite grains, sometimes together with other minerals.
Dripstone formations that rise upward from the floor of caves.
Dripstone formations that taper downward from the ceilings of caves.
A deposit of calcium carbonate formed by evaporation of water in caves
A metal in group 1 of the periodic table, having one electron it its outer electron shell
Group 17 of the periodic table. Elements with 7 electrons in their outer shell.
The mineral form of table salt (NaCl)
Minerals or rocks produced by
precipitation of minerals from high-salinity water (such as seawater) during evaporation
Minerals containing oxidized sulfur in the form of sulfate ions with the formula SO₄²⁻
A crystal form of gypsum that is transparent and colourless.
A crystal form of gypsum with long fibres.
Minerals containing negative ions of reduced sulfur.
The state of an atom when an additional electron is added during a reaction; opposite of oxidation
A change in sediment after it is deposited
High-quality reflection of light from the surfaces of a mineral.
A solid shape with twelve faces
An element has different oxidation states when it can lose or share different numbers of electrons to form ions
Iron that has lost two electrons from each atom. Formerly known as ferrous iron
Iron that has lost or shared three electrons from each atom; oxidized iron. Formerly known as ferric iron.
Layers of iron-rich minerals and chert that were particularly common in the Proterozoic Eon
A black variety of hematite with a highly reflective metallic lustre
Minerals or other substances composed wholly of a single element.
Mineral deposits formed at the Earth's surface by sedimentary processes, typically the concentration of dense minerals by water flow.
The practice of shaking sediment grains in water to separate gold and other other high-density minerals.
A type of lustre in which different wavelengths of light are refracted differently, typical of diamond