5 Atmospheric Circulation
This chapter is a pre-review version
Learning Outcomes
By the end of this chapter you should be able to:
- Explain pressure gradient flow, the Coriolis effect, and how they interact;
- Describe the large-scale features of atmospheric circulation.
Energy balance and atmospheric flow
Energy balance
If we compare the distribution of incoming solar energy with the energy that returns from the Earth into space, we see that there is a large difference between the two curves. In equatorial regions, the incoming energy is much greater than the outgoing energy, whereas at the poles, more energy is radiated than is received.
Therefore, there must be continuous redistribution of energy from the equator toward the poles. This redistribution is achieved by circulation of air in the Atmosphere and of water in the Hydrosphere. In this section we examine the circulation of air, and what it means for those on the surface of the Earth.
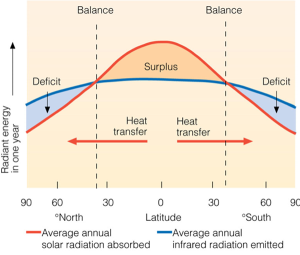
From: https://www.e-education.psu.edu/earth540/content/c4_p2.html
Pressure-gradient flow
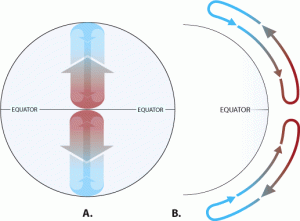
To understand the flow of air and energy in the Earth, it’s helpful first to consider a hypothetical planet that is not rotating, but which nonetheless receives heat from all sides at the Equator just like the Earth. Such a planet is shown in the diagram.
On this planet, as on Earth, air is intensely heated near the equator. Warm air expands, decreases in density, generating a region of low pressure. The lower-density air is buoyant and rises. The rising air cools adiabatically; clouds may form after it reaches its dew point. The rising air raises the tropopause and spreads out N and S of the tropics. As the air cools, its density increases and it eventually flows back to the surface forming a region of high pressure near the pole. At ground level, air flows from high pressure to low pressure. This is called pressure-gradient flow. The zone of low pressure and clouds near the equator is the intertropical convergence zone (ITCZ).
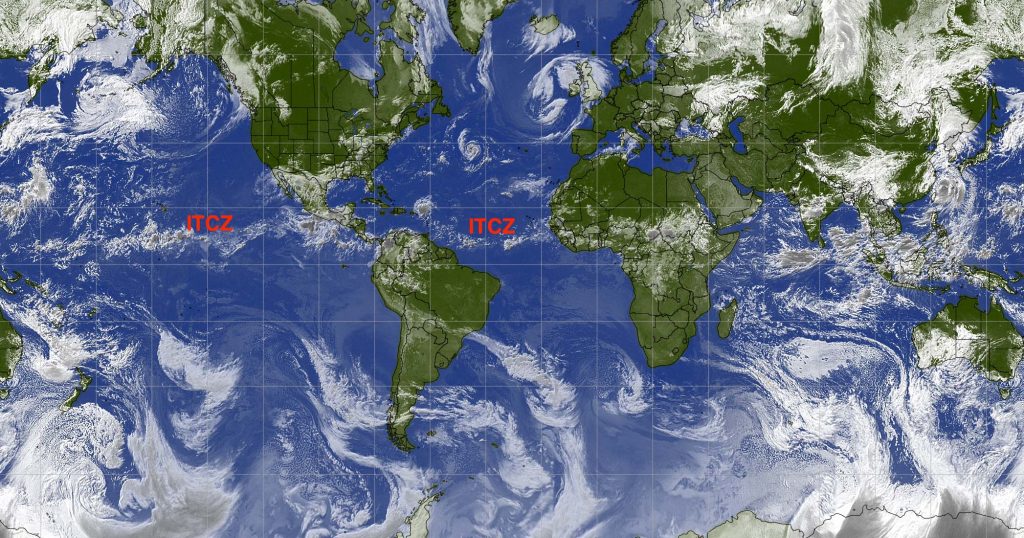
Wind speed is controlled by the pressure gradient. On the idealized non-rotating Earth, the wind follows the pressure gradient, and flows at 90° to the isobars (lines of constant pressure). If isobars are far apart, the pressure gradient is small, and the winds are slow. If isobars are close together, the pressure gradient is steep, and wind speed is higher.
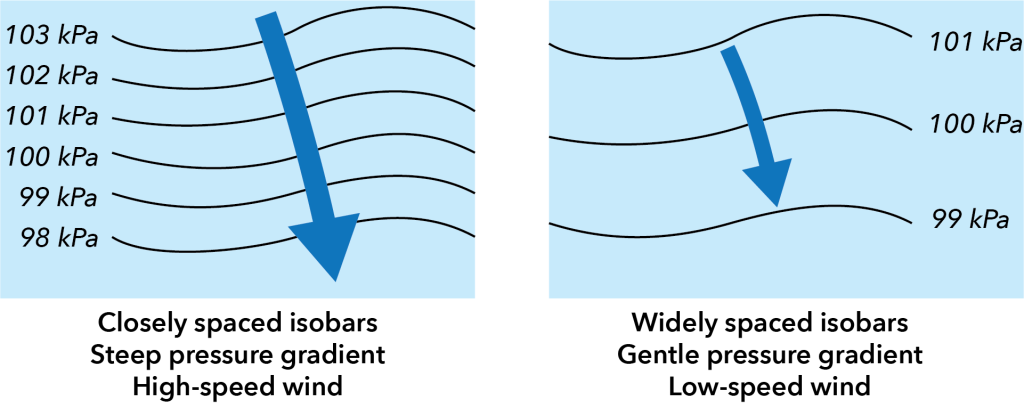
Rotating Earth: Coriolis effect
On the real Earth, pressure gradient flow behaves differently, because rotation causes the Coriolis effect. The Coriolis effect works because of a principle in physics called conservation of momentum (the momentum of a body is the product of its mass and its velocity).
The Equator is far from Earth’s spin axis; air that appears stationary is actually moving fast from W to E because of Earth’s spin. Arctic regions are close to the Earth’s axis; Earth’s spin moves air more slowly near the poles. Momentum is conserved as air masses move around the Earth’s surface.
Therefore, air that moves from the Equator toward the North Pole carries extra eastward momentum, which causes it to get ahead of the Earth’s rotation. It appears to be deflected to the east. Air that moves from the pole toward the Equator carries less momentum. It tends to lag behind Earth’s rotation. It appears to be deflected to the west. Notice that in both cases the air is deflected to the right, relative to its starting direction.
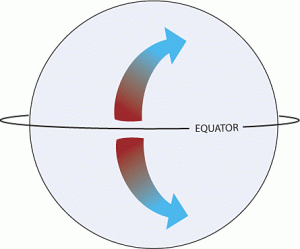
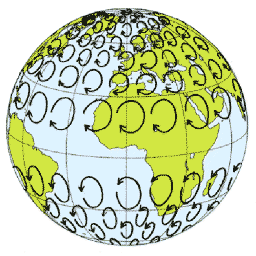
Air travelling east and west, or any other direction, is also subject to Coriolis deflection. For example, east-flowing air tends to continue in a straight line, while the Earth turns counter-clockwise. Eventually that straight line travel would bring it back toward the Equator; to an observer on the Earth’s surface the path of the air has been deflected to the right.
Mathematically, these two explanations are equivalent. Any moving material that travels north of the Equator appears, to an observer standing on the rotating Earth, to be deflected to the right. In the southern hemisphere the deflections are to the left. The Coriolis effect is responsible for all the atmospheric swirling patterns visible in satellite photos of the Earth.
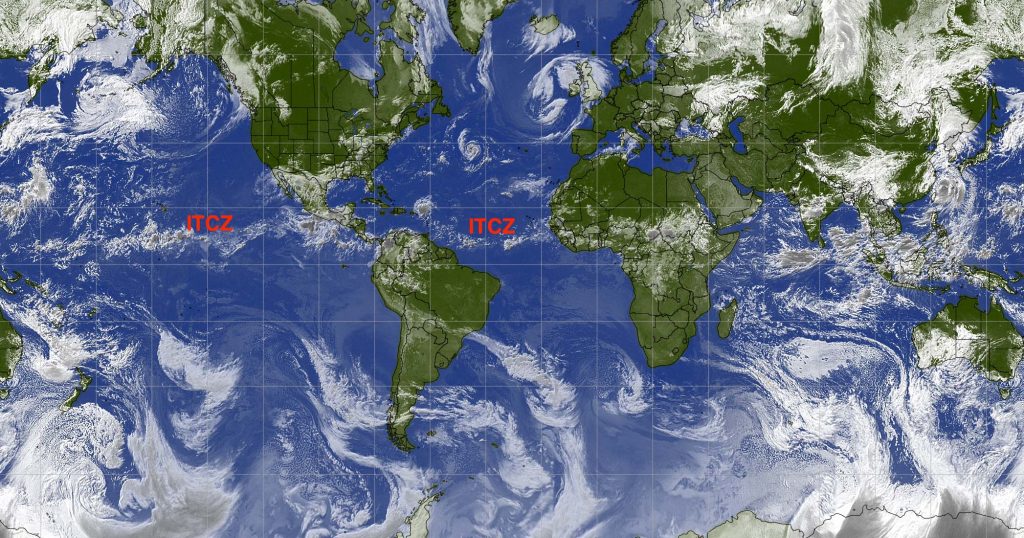
For short distances of travel, Coriolis deflections are undetectable. The Coriolis effect (contrary to popular belief) has no impact on the motion of water in a bathtub or toilet. It’s a major factor in the motion of material that travels hundreds or thousands of kilometres.
Hence, high-level winds leaving the ITCZ are deflected to the east, whether in the northern or southern hemisphere, and low level returning winds are deflected to the west.
Global atmospheric circulation
Because of this deflection, air that leaves the ITCZ high in the atmosphere, travelling north, never makes it to the North Pole. It is deflected to the right until it is travelling approximately eastward, parallel to the isobars (lines of equal pressure). At that point, it is possible to show mathematically that the pressure gradient acting to pull the air northward is exactly balanced by the Coriolis deflection to the south, and the air flow stabilizes parallel to the isobars. Such a flow is called geostrophic flow.
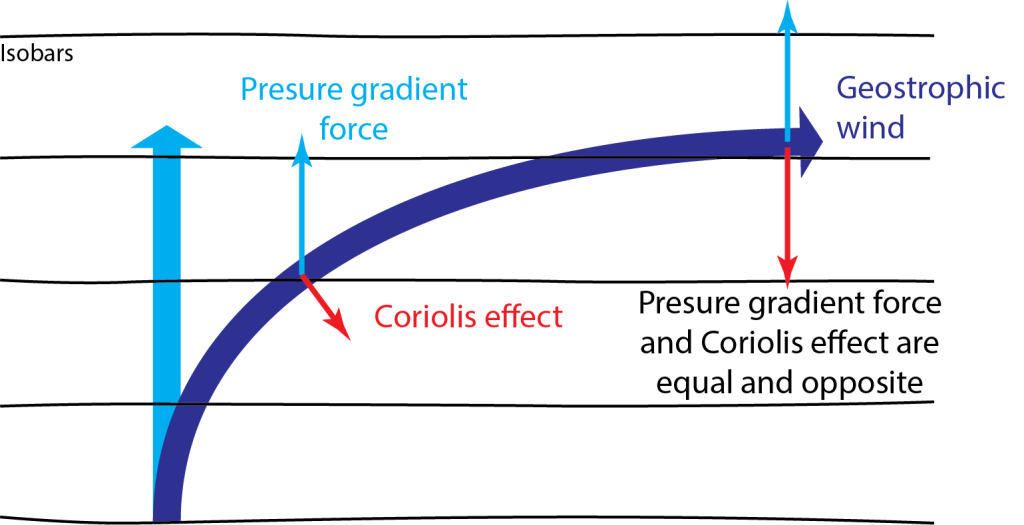
Because of the coriolis deflection, pressure gradient flow from the ITCZ reaches only ~30° N and S of the Equator before it starts to descend in a belt of high pressure known as the ”subtropical high” or “the horse latitudes”. (The origin of this name is uncertain.)
Air descends towards ground level in the subtropical high, and pressure gradient flow forces it back towards the Equator. The Coriolis effect deflects it towards the west, forming tropical easterlies [1].
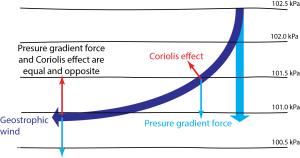
The belt of circulation between the ITCZ and the horse latitudes is called the Hadley Cell
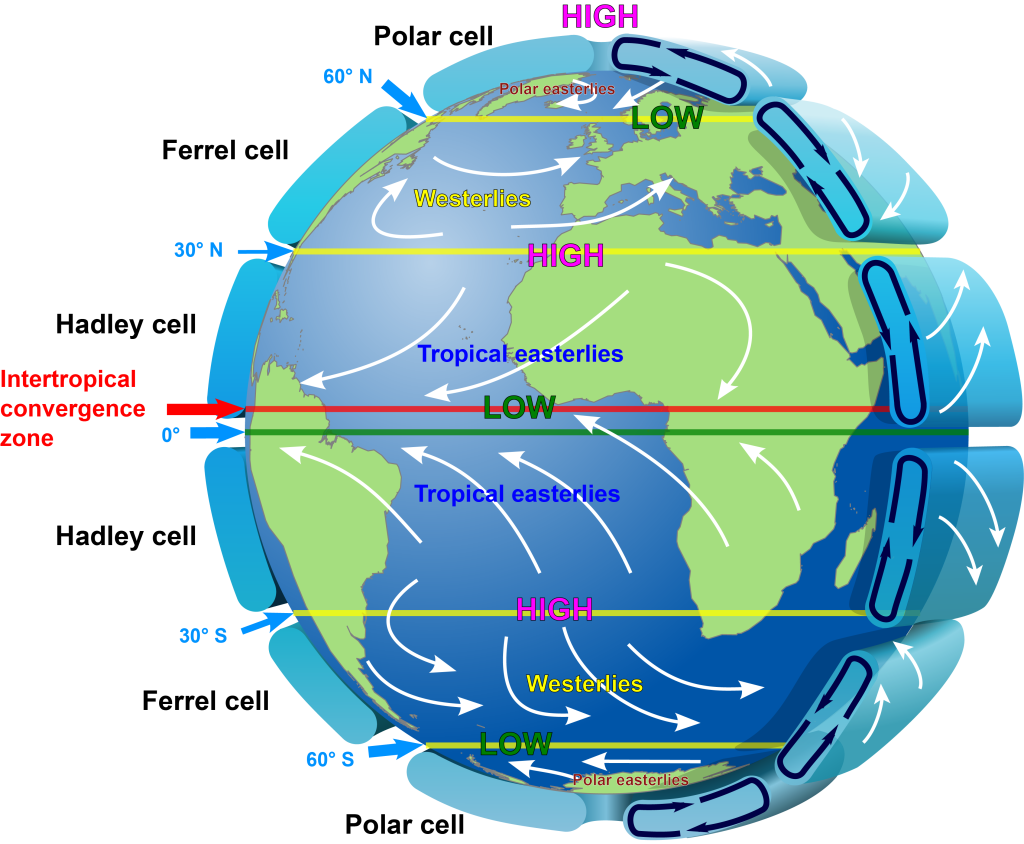
To the north of the Hadley Cell, warm air accumulates in a belt around 60° from the Equator, producing another belt of low pressure. Along this belt, the warm air from the tropics meets cooler air flowing from the pole. The belt is known as the polar front. (A front is a boundary between warm and cold air masses.) Warm air rises at this belt and flows towards the pole before descending at the polar high pressure area. Low altitude winds blow away from the pole and are deflected toward the west by the Coriolis effect. Because mariners name winds according to the direction they come from, not the direction they blow towards, these winds are known as polar easterlies.
Between the horse latitudes and the polar front a third circulation cell operates, in the opposite direction to the Hadley and Polar cells. Flow at low altitudes in the Ferrel cell is toward the pole, and winds are deflected towards the east by the Coriolis effect. Because mariners name winds according to the direction they come from, not the direction they blow towards, these prevailing mid-latitude winds are known as mid-latitude westerlies or just [pb_glossary id=”3064″]westerlies[/pb_glossary].
West-to-east winds also prevail at high altitude above the polar front. There, a belt of geostrophic winds called the jet stream follows a pressure gradient that marks a slope in the tropopause.
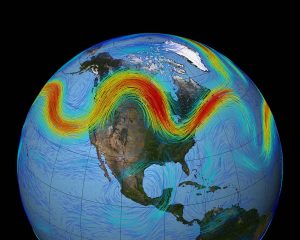
The position of the polar front (where Ferrel and Polar cells meet) is unstable. This can lead to huge undulations in the position of the polar front, called Rossby waves. Rossby waves affect the position and velocity of the jet stream. They can cause cold air to move towards the equator and warm air to move toward the poles. They can ‘block’ air masses, stalling them over certain regions. We will consider these air masses next.
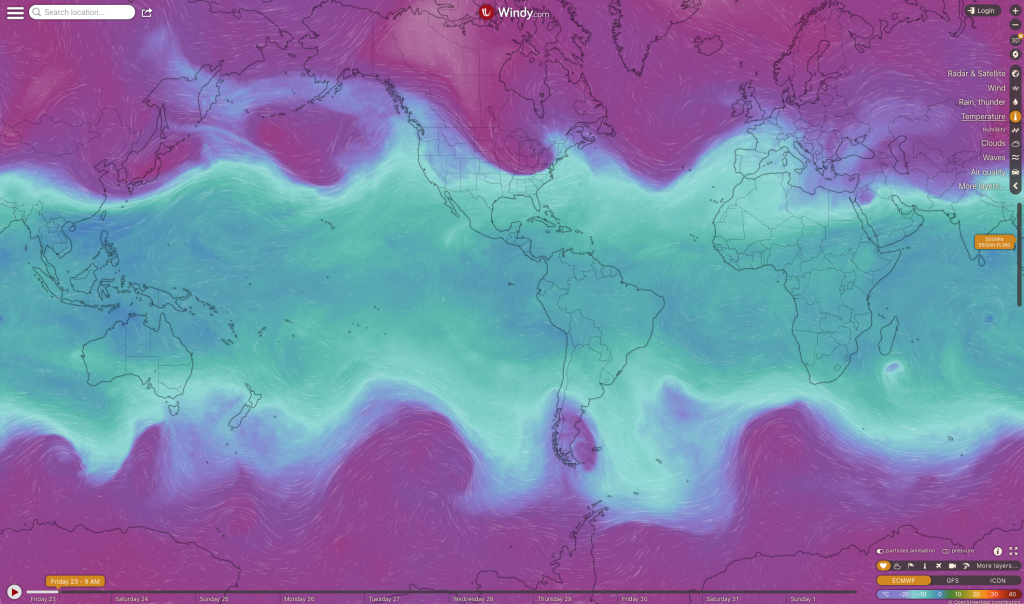
Air masses and fronts
Air masses
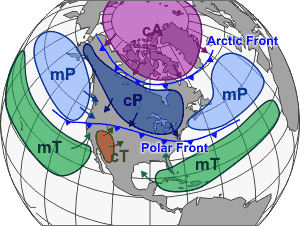
Weather is controlled by air masses, which are large volumes of air that have internally consistent properties, including pressure, humidity, and temperature. Air masses commonly meet at fronts.
Air masses can be categorized by location of origin…
- c = continental
- m = maritime
…and latitude of origin
- A = arctic
- P = polar
- T = tropical
For example, an air mass developed over western Canada would be designated cP, for “continental polar”.
Fronts
Where air masses of different temperature meet, fronts develop. Heavy rain or snow, and rapid changes of wind direction may occur at a front.
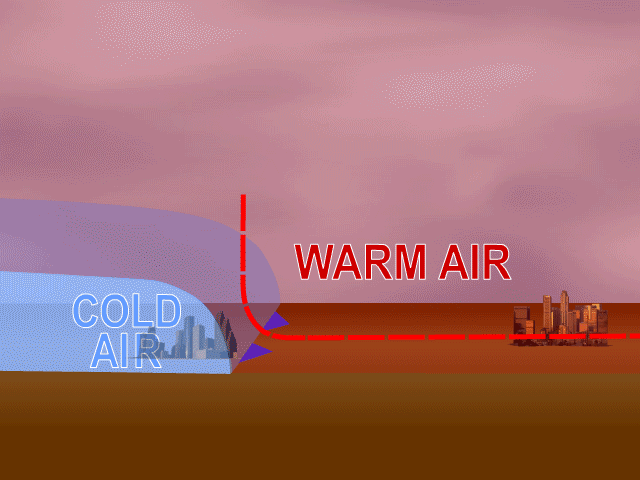
At a cold front, cold air advances into an area previously occupied by warmer air. The cold air, being denser, slides under the warm air and pushes it upwards, prompting adiabatic cooling and precipitation. Cold fronts may be marked by a steep wall of cumulonimbus cloud that may rise to the tropopause.
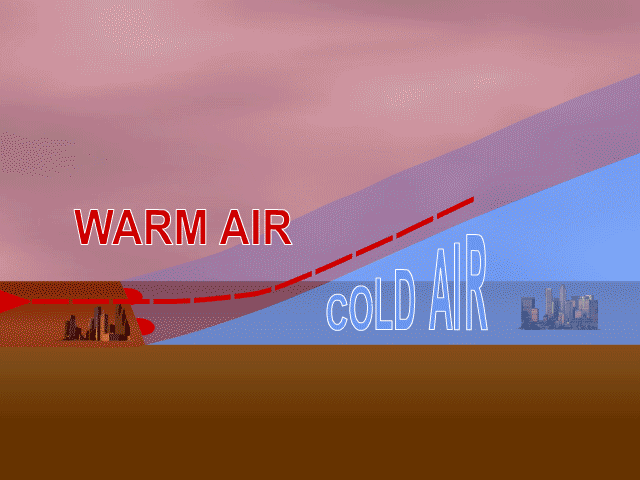
At a warm front, warm air advances into an area previously occupied by colder air. The warm air rises over a wedge of cold air producing clouds and precipitation, but the cloud ceiling descends gradually as the front approaches. Horizontally extensive stratus and nimbostratus clouds are common. In a chapter on weather we will look at cloud types in more detail.
- These winds are also known as trade winds because of their importance to European colonial sailing ships. In recognition of the fact that the “trade” included the forcible transport of many millions of humans from Africa to the Americas, we prefer the term “tropical easterlies” ↵
A change to a system is adiabatic no heat energy is gained or lost during the change
For an undersaturated body of air, the dew point is the temperature at which the air would become saturated. The dew point is always equal to or lower than the actual temperature of the air.
The boundary lying between the troposphere and the stratosphere, where temperatures reach a minimum after decreasing with altitude in the troposphere, and before increasing once again in the stratosphere.
The flow of air from a region of high pressure to one of low pressure.
A low pressure band in the Earth’s atmosphere lying approximately along the equator
The apparent deflection of objects travelling over the surface of a rotating sphere, because of different rotation speeds at different latitudes
A principal in mechanics in which the product of mass times velocity is conserved
Persistent east-to-west winds that flow in the tropics
A circulation of air that first rises near the equator before cooling and descending at the "horse latitudes"
A low-pressure belt where warm air from the tropics and cold air from the pole converge, typically producing cloud and preciptitation
Low altitude polar winds that move air from east to west and towards the polar front.
A feature of atmospheric circulation at moderate latitudes; air flows towards the equator at low altitude and away from the equator in the upper troposphere.
West-to-east winds moving at the top of the troposphere, following a pressure gradient. The jet stream typically meanders, and roughly follows to the polar front.
Large-scale undulations of the polar front and the jet stream
A border between air masses of different temperatures; usually associated with precipitatoin and changes in wind direction.
A boundary between cold and warm air where the cold air is advancing into the area previously occupied by warm air. The denser cold air pushes the warm air upwards, causing precipitation.
Towering clumped rain-producing cloud, typically terminated at the top with an anvil-shaped ice-cloud. Typically produces lighning and thunder.
A boundary between air masses where warm air advances towards and over cold air
Clouds that form horizontal, flat layers at low altitudes. Typical of warm fronts.
Horizontal low-altitude cloud layers (stratus) that produce steady precipitation.